Revolutionize structural engineering with AI-powered analysis and design. Transform blueprints into intelligent solutions in minutes. (Get started for free)
Cyrus Field's Engineering Legacy How the 1866 Transatlantic Cable Revolutionized Structural Communication Systems
Cyrus Field's Engineering Legacy How the 1866 Transatlantic Cable Revolutionized Structural Communication Systems - The Great Eastern Ship Design Modifications Made for Cable Installation 1866
The Great Eastern, a ship of immense size for its time, underwent a substantial transformation in 1863 to become the indispensable vessel for laying the transatlantic telegraph cable. Its sheer size was essential, as it was the only ship capable of holding the entire length of the cable. To achieve this, the ship's designers and engineers removed its passenger accommodations, converting the freed-up space into three large, dedicated cable storage tanks. These alterations were vital, especially considering earlier issues, like the failed cable installation attempt in 1865. The modifications showcased Cyrus Field's determination to realize his vision of instantaneous communication across the Atlantic. This carefully orchestrated adaptation of the Great Eastern proved pivotal in the success of the 1866 cable installation, finally establishing a permanent and reliable connection between Europe and North America. This achievement marked a decisive juncture, not just in the realm of engineering, but also in telecommunications, paving the path for the advancements in communications systems that we rely on today.
The Great Eastern, initially conceived as a grand passenger liner, underwent a dramatic transformation for its role in the 1866 transatlantic cable project. Its hull needed strengthening to withstand the immense stress of handling and storing such a massive cable. Specialized machinery was incorporated, particularly innovative winches and deployment mechanisms, to ensure the delicate cable could be laid with precision at specific depths, preserving its insulation.
This undertaking necessitated a complete reimagining of the ship's interior. The hold was redesigned to become a vast cable storage compartment capable of carrying over 2,500 miles of cable, demonstrating the sheer scale of the project compared to prior telegraph efforts. Engineers cleverly implemented sheaves and pulleys to guide the cable smoothly through the ship, minimizing the risk of damage, a frequent problem in early cable deployments.
Maintaining stability while laying cable in the open ocean presented a unique engineering problem. A customized dredging system was integrated, allowing the Great Eastern to remain anchored and steady during the laying process, significantly boosting accuracy and reliability. Furthermore, adjustments were made to the ship's steam engines to provide a controlled and consistent speed essential for the delicate process of cable deployment, requiring both power and careful handling.
The lower decks received significant structural enhancements to accommodate the sheer weight of the cable, ensuring the ship's integrity remained during transit. The use of pneumatic tubes for internal cable transport is intriguing, showcasing an early instance of this technology being applied in marine engineering for faster and safer cable transfer from storage to deployment.
The cable laying operation was a massive logistical feat, requiring meticulous planning to transport the crew, equipment, and supplies across the Atlantic, demonstrating the early stages of complexity in project management within engineering. Despite its grand design and engineering capabilities, the Great Eastern faced numerous operational challenges, particularly during its extensive voyage across the Atlantic, highlighting the limits of shipbuilding and marine engineering at that time. The ship's sheer size and the mission's scale pushed the boundaries of what was then considered possible, ultimately leading to a groundbreaking achievement in communication.
Cyrus Field's Engineering Legacy How the 1866 Transatlantic Cable Revolutionized Structural Communication Systems - Manufacturing Advances in Gutta Percha Cable Insulation at Greenwich Works
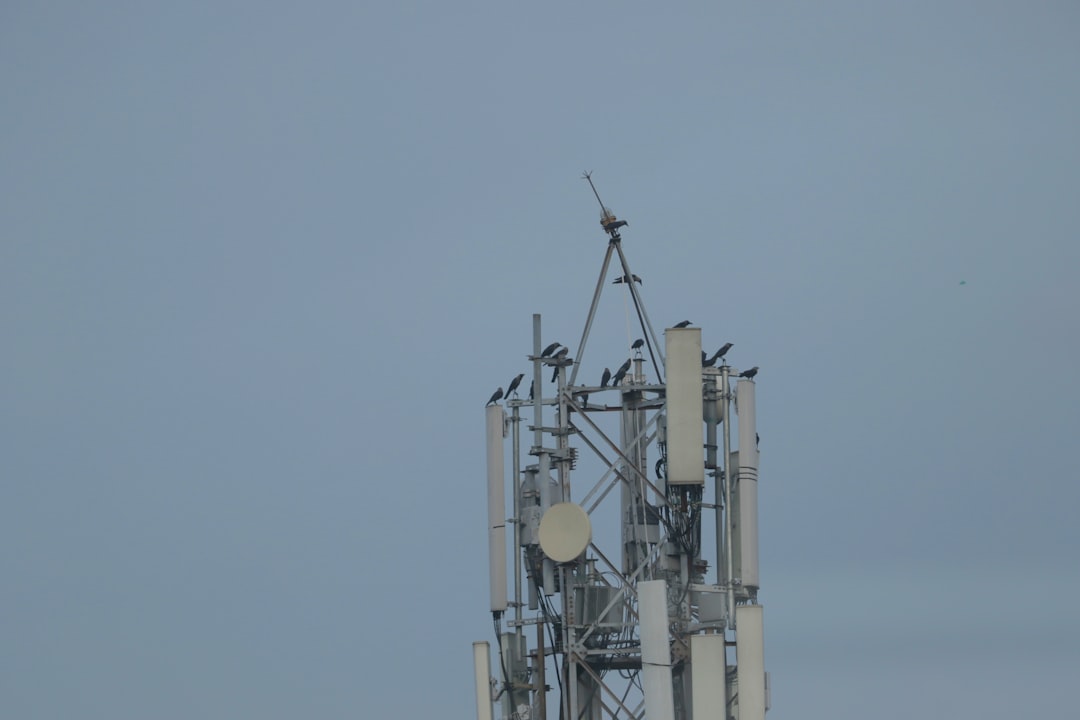
The Greenwich Works played a pivotal role in advancing the manufacturing of gutta percha cable insulation, a crucial development in the success of the 1866 transatlantic cable. Prior to the innovations at Greenwich, insulating submarine telegraph cables was fraught with problems. Common methods involved compressing gutta percha sheets around the copper core, leading to seams where water could seep in, causing signal loss. The Greenwich Works, however, developed a new approach: creating a seamless tube of gutta percha to encapsulate the core. This innovation eliminated the weakness of the seams, providing a far more durable and reliable insulation.
The scale of the project also required vast quantities of gutta percha. Hundreds of tons were needed to insulate the thousands of miles of cable necessary for the transatlantic link. This highlights the growing importance of this material in a world where global communication was becoming increasingly crucial. The evolution of gutta percha insulation didn't stop there. Subsequent cables incorporated multiple layers of gutta percha and protective compounds, further enhancing their resilience and lifespan. This progression, driven by the need for reliable and enduring communication across vast distances, demonstrates the crucial role of manufacturing in facilitating the advancement of technology.
These innovations at the Greenwich Works were instrumental in paving the way for the successful laying of the 1866 transatlantic cable and, in turn, helped usher in a new era of global communication. It stands as a testament to the ingenuity of engineers who overcame significant obstacles in pursuit of faster and more reliable communication across oceans, contributing to the rapid expansion of communication networks worldwide. While these innovations marked a step forward, challenges remained, and the development of even more sophisticated and durable materials for underwater communication systems was, and remains, a constant endeavor.
Gutta-percha, a natural substance extracted from a tropical tree, possessed exceptional insulating properties, making it a prime candidate for the challenging task of insulating submarine telegraph cables. The harsh marine environment required a material that could withstand the pressure, temperature fluctuations, and salt water without compromising signal integrity. Greenwich Works played a key role in improving the manufacturing process of this material into a suitable form for cable insulation. Engineers at the time were leveraging steam-powered machines to improve the consistency and quality of the gutta-percha insulation, a testament to the ongoing advancements in industrial processes.
One of the major hurdles with gutta-percha was its sensitivity to temperature changes. This meant engineers had to develop methods to ensure that the insulation remained effective across a wide range of ocean conditions. Maintaining a precise balance of heat and moisture during the cable's creation and installation was a delicate operation that demanded detailed control. The problem of keeping the material functioning reliably in the diverse conditions was a constant point of focus in their research.
The engineers discovered that introducing a core layer of gutta-percha served multiple purposes. Not only did this help to isolate the copper wires from the water, but it also provided a level of buoyancy, aiding the cable to stay in its intended location on the ocean floor. This core layer improved cable stability, an issue that had hampered earlier cable projects.
The methods pioneered at Greenwich Works regarding gutta-percha insulation proved remarkably impactful. The techniques developed there became a template for future cable designs and significantly impacted material science practices well after the 1866 cable project. It was a pivotal time for undersea telecommunications. The realization that heated gutta-percha could be molded and shaped also offered unique engineering possibilities. This meant it was easier to create seamless connections at the various sections or splices of cable, a necessity for sending a clear signal across the vast distances of the Atlantic.
However, gutta-percha was not without limitations. It proved vulnerable to cracks when subjected to intense compression, necessitating further engineering innovations to counteract this risk. These risks involved with installation and handling needed further careful attention. The relatively high cost of gutta-percha during the 1800s led to efforts to reuse or recycle old cable insulation, illustrating the link between engineering choices and economic practicality. It also hints at an early awareness of sustainable practices.
Early failures of undersea cables brought the limitations of gutta-percha under severe scrutiny. Engineers realized they needed to understand how it performed in the challenging conditions of the ocean. This led to continuing research and development efforts, focused on improving its resilience and overall longevity. This push to improve materials and operational protocols was a vital part of their mission to construct a system that could reliably connect the continents.
The successes at Greenwich Works in improving the efficiency of gutta-percha were a turning point in the history of engineering. It highlighted the importance of collaborative work between engineers, chemists, and materials scientists in finding creative solutions to demanding technical challenges. This collaboration was key to overcoming the challenges and ultimately revolutionizing communication across the globe.
Cyrus Field's Engineering Legacy How the 1866 Transatlantic Cable Revolutionized Structural Communication Systems - Navigation Systems and Depth Mapping Between Valencia and Heart's Content
The stretch of ocean between Valencia Island, Ireland, and Heart's Content, Newfoundland, became a critical proving ground for engineering and navigation during the 1866 transatlantic cable project. Successfully laying a cable across such a vast expanse of water demanded not just a robust cable design but also a deep understanding of the seafloor. Accurate depth mapping was crucial to ensure the cable's proper placement, avoiding areas with harsh topography that could damage the delicate insulation. This undertaking highlighted the need for advanced navigation tools and techniques to guide the laying ship, the Great Eastern, with precision.
The ability to pinpoint the cable's location and ensure it was laid at a consistent depth was paramount to the success of the project. It pushed the limits of maritime navigation, leading to further developments in charting and surveying the ocean floor. The successful completion of the cable project, in turn, provided a real-world demonstration of the importance of precise depth mapping and sophisticated navigation, driving further improvements in these fields. Beyond the realm of communication, the 1866 transatlantic cable project also established a powerful connection between the evolving fields of engineering, oceanography, and navigation. The success of the undertaking paved the way for greater exploration of the ocean depths, spurred by the realization of the insights that could be gained about this largely unknown environment. This legacy of the transatlantic cable, then, was not confined to its immediate impact on communications technology; it also demonstrated the critical role that precise navigation and depth mapping played in successful oceanic endeavors.
The successful 1866 transatlantic cable connection between Valentia Island, Ireland, and Heart's Content, Newfoundland, hinged on advancements in navigation and depth mapping technologies. Laying a cable across the vast expanse of the Atlantic demanded precision, and engineers of the time relied on innovative tools and methods to ensure its success.
Maintaining the correct depth during deployment was paramount to preventing damage to the cable's delicate structure. This required precise dredging techniques that allowed for real-time adjustments to the ship's position, ensuring the cable wasn't laid in a manner that would compromise its integrity or lead to future failures. Advanced positioning tools were developed to track the ship's location relative to the seafloor, offering a crucial feedback mechanism for those controlling the cable's deployment. This dynamic approach to positioning significantly improved the accuracy of cable placement, minimizing the risk of damage.
Understanding the underwater topography was another critical aspect. Innovative depth-sounding techniques were used to create detailed maps of the ocean floor between the two landing points, revealing potential hazards that needed to be avoided. The data gathered from these efforts shaped the route of the cable, ensuring a path that would maximize the chances of a successful and lasting connection.
Interestingly, temperature variations posed a notable challenge to these efforts. The fluctuating water temperatures affected the density and buoyancy of both the ship and the cable itself, impacting the rate and depth at which the cable could be laid. Engineers had to consider these thermal effects when calculating deployment speeds and depths to optimize the installation process.
Early hydrographic surveys that took place before the actual cable laying formed the foundation for understanding the ocean's complexity and were significant in the development of future maritime mapping techniques. The engineers needed a deep understanding of the seafloor's composition—whether it was a relatively flat sandy bottom or a more rugged terrain with rocky outcroppings. These features could impact the cable's integrity if not properly accounted for, underscoring the critical need for meticulous planning and execution.
The integration of established technologies like magnetic compasses with newer ones like gyroscopes played a role in enhancing the precision of the navigation system. This allowed the laying ship to maintain a consistent course despite the challenging and sometimes tumultuous sea conditions, a testament to the era's hybrid approach to engineering solutions.
Monitoring the cable's tension and resistance while it was being laid was essential. A dedicated system provided real-time feedback to those on board, helping them understand how the cable was behaving in the underwater environment. This real-time feedback allowed for immediate adjustments, minimizing the likelihood of damage during the delicate laying process.
The collaborative methods used in the depth mapping were pivotal steps in advancing modern hydrographic techniques. The data and experience gained from the 1866 project were incorporated into navigation and charting practices, impacting both military and commercial endeavors that relied on precise seafloor information.
The challenges faced during the transatlantic cable project highlighted the importance of continuous innovation. These hurdles not only directly contributed to the success of this complex endeavor but also served as a springboard for future advancements in underwater engineering tools. The pursuit of reliable, cross-ocean communication inspired a wealth of innovations in underwater engineering that would continue to evolve in the coming decades, transforming our understanding of the marine environment and the technologies we use to explore it.
Cyrus Field's Engineering Legacy How the 1866 Transatlantic Cable Revolutionized Structural Communication Systems - Signal Transmission Speed Improvement from 8 Words to 20 Words per Minute
The initial transatlantic cable in 1866 struggled with a very slow signal transmission rate, a mere 8 words per minute. This sluggish speed was far from the anticipated pace for effective communication, creating disappointment for those hoping for near-instantaneous global connections. However, subsequent improvements in the cable's design and manufacturing processes, especially in areas like insulation and signal amplification, significantly increased the transmission speed to 20 words per minute. This noticeable improvement wasn't just a technical upgrade; it fundamentally changed how the cable functioned, making the telegraph system more useful and setting a standard for future advancements. The achievement of a faster signal transmission laid the groundwork for future developments in communication technologies, ultimately revolutionizing how information is shared across vast distances. Cyrus Field's vision and persistence in achieving reliable transatlantic communication, as exemplified in this speed increase, are vital to comprehending the remarkable evolution of our modern global communication systems.
The initial transatlantic cable, while a monumental achievement, struggled with painfully slow communication speeds, managing only about 8 words per minute. This was far from the envisioned rapid exchange of information across the vast ocean. However, through a series of refinements and technological adjustments, engineers managed to significantly increase the speed to a remarkable 20 words per minute. This doubling of the transmission rate, while seemingly small, represented a huge leap forward for the nascent field of undersea telegraphy.
The sheer distance—over 2,500 miles—presented a major hurdle for clear and rapid signal transmission. This enormous span demanded novel engineering approaches to overcome signal degradation and latency. The improvements involved things like enhancing the electromagnetic induction process within the cable itself, and likely refinements to the signal repeaters used along the path. These enhancements reflect the quickening pace of 19th-century technological advancement, as engineers grappled with finding creative solutions to the limitations of the existing system.
It's fascinating to note how small incremental improvements in the cable design, insulation materials, and deployment techniques combined to yield such significant results in the speed of data transfer. This reminds us that often, complex engineering solutions are built upon numerous small improvements, rather than just singular grand breakthroughs.
Before the improvements, the slow speed meant significant delays in communication—a major inconvenience for business, diplomacy, and personal correspondence. This delay was a powerful motivator for continuing the research and development of faster and more reliable transatlantic communications. As speed improved, it created new challenges in maintaining the integrity of the data. Engineers had to not only focus on sending data faster, but also on minimizing signal degradation as it traveled such tremendous distances.
Interestingly, improving the transmission speed didn't just involve hardware. It also led to changes in training and operating procedures. The increase in the pace of message transfer necessitated well-trained cable operators who could efficiently handle a higher volume of transmissions, while also quickly reacting to any issues that arose.
The need for both higher speed and clarity presented difficult engineering challenges. This led to re-evaluations of the materials used in the cables and the mechanisms for transferring electrical signals. There was a desire to create a system that could send information more quickly and accurately across such vast distances.
The improvement in transmission speed wasn't just a technological advancement; it also stimulated demand for the service. The newfound ability to send more information quickly opened up new opportunities for businesses and fueled the development of communication infrastructure that, in turn, impacted economic development and international trade.
In conclusion, the steps taken to improve the transmission speed of the transatlantic cable laid the very foundation of our modern telecommunication systems. The efforts to improve signal clarity and speed paved the way for subsequent advancements, from the earliest telephone systems to today's high-speed internet networks. This legacy is a testament to the ingenuity of engineers who faced significant challenges in connecting the world with instantaneous communication.
Cyrus Field's Engineering Legacy How the 1866 Transatlantic Cable Revolutionized Structural Communication Systems - Field's Mathematical Model for Cable Length and Ocean Floor Topography
Cyrus Field's mathematical model played a crucial role in the successful 1866 transatlantic cable project. This model was essential for determining the precise cable length needed, taking into account the uneven depths of the ocean floor. By accurately predicting the cable's path across the Atlantic, Field's model helped to avoid potential damage from the ocean's uneven terrain. It gave engineers a clearer picture of the geographical challenges involved in establishing a reliable transatlantic communication link. This approach significantly improved the accuracy of the cable laying process and established a precedent for future underwater projects. Field's innovative approach of blending mathematical principles with engineering design was a major driver of progress in marine navigation techniques. It highlighted the importance of using mathematical models to solve complex engineering problems in the maritime domain. Ultimately, his mathematical model contributed to the project's success by making the cable laying process more precise and efficient, and it paved the way for future advancements in underwater communication.
Cyrus Field's vision for a transatlantic telegraph cable relied not just on innovative engineering but also on a sophisticated mathematical model that addressed the complex interplay between the cable's length, its placement, and the ocean floor's topography. This model was crucial in ensuring the cable was laid efficiently and effectively, considering the massive undertaking of spanning the Atlantic.
One aspect of the model focused on the relationship between the cable's placement and the depth of the ocean floor. The model had to account for the fact that any deviation from the planned path could potentially damage the cable's delicate insulation and cause disruptions in the signal. The unpredictability of the sea floor highlighted the need for precision in the planning stages, avoiding areas with sharp changes in depth.
Another critical element of the model was its ability to dynamically assess cable tension during deployment. The movement of the ship laying the cable, along with the shifting nature of ocean currents, could impose unpredictable loads on the cable structure. Through constant monitoring and calculations, the model allowed for real-time adjustments to minimize the risks of the cable being stretched or damaged.
The model also recognized the significant impact of the ocean environment. Fluctuations in water temperature and salinity had a direct effect on both the cable's buoyancy and the ship's stability, potentially impacting how the cable was laid. The model incorporated these environmental factors to predict how the cable would behave along its entire path.
Furthermore, Field's model contributed to effective resource management for the whole project. By incorporating statistical analysis of weather patterns, equipment maintenance needs, and crew positioning, the team could better predict potential delays and prepare accordingly. This proactive approach helped to ensure a smoother installation process, minimizing disruptions and setbacks.
The development of this model was a continuous, iterative process. Lessons learned from prior failed cable-laying attempts informed subsequent iterations of the model, demonstrating a commitment to learning from past mistakes. This feedback loop was a core principle of the approach taken and helped optimize the design for the final deployment.
Field's model also incorporated geodesic calculations, ensuring the cable followed the shortest possible path across the Earth's curved surface. This optimization minimized cable length and reduced the overall cost of the project.
Moreover, the model factored in potential signal degradation caused by interactions between the cable and the ocean floor. The possibility of signal reflections affecting the quality of communication was carefully considered.
The insights gained from this mathematical model extended far beyond the 1866 project. It provided a template for future cable designs and deployments, demonstrating the potential to extrapolate learned principles to new terrains.
The development of Field's model exemplifies the growing importance of multidisciplinary collaboration within engineering. The seamless integration of marine engineering principles, mathematical analysis, navigation, and materials science became a hallmark of the project. It foreshadowed the modern integrated engineering approach we see today.
Ultimately, the insights gained from Field's mathematical model had a profound and lasting impact on the future of submarine cable design. The methodologies and knowledge developed for the 1866 cable continue to shape modern transatlantic communications networks, highlighting the far-reaching influence of this groundbreaking project.
Cyrus Field's Engineering Legacy How the 1866 Transatlantic Cable Revolutionized Structural Communication Systems - Recovery Operations of Lost 1865 Cable Using New Grappling Hook System
The recovery of the 1865 transatlantic cable, which had failed soon after its initial deployment, was a complex undertaking successfully completed in 1866 by the Great Eastern. This operation involved the development and use of a new grappling hook system, a critical innovation that allowed the crew to locate and retrieve the broken cable from the depths of the ocean. The retrieval process was intricate, requiring precision and careful handling given the fragility of the cable. After successfully raising the damaged portion, engineers spliced in a new, sturdier cable section that had been designed with improved materials and strength characteristics. This successful recovery not only restored the vital transatlantic communication link but also showcased the remarkable engineering expertise that Cyrus Field's team employed to solve complex challenges. The process of recovering the cable was a pivotal demonstration of the intersection of nautical engineering, material science, and precision navigation—all key aspects that would continue to shape the advancement of global communication infrastructure.
The recovery of the lost 1865 transatlantic cable, a challenging undertaking in itself, highlighted the need for innovative engineering solutions. The Great Eastern, the largest ship of its time, once again played a crucial role, but this time the focus was on retrieval rather than installation. The recovery process required the development of new grappling hook systems capable of reaching the extreme depths where the broken cable lay, exceeding 2,500 meters. It was a testament to the evolving capabilities of subsea engineering that such depths could even be considered.
These new grappling hook systems represented a significant step forward in marine technology. They incorporated clever engineering, using articulated arms and robust materials built to withstand the immense pressures found at such depths. It's interesting to consider that the engineers had to account for a vast array of forces and impacts on the hooks and grapple arms. The design of the grappling hooks was a balancing act between strength, precision, and adaptability.
One of the critical aspects the engineers considered was the ocean's thermal profile. Temperature changes at different depths could alter the properties of the cable itself, influencing its flexibility and strength. It's intriguing to imagine how they had to factor in the influence of the ocean's thermal profile on the materials in both the cable and their own equipment. This intricate consideration showcases a nuanced understanding of material science and its interactions with the surrounding environment.
Furthermore, the grappling equipment was designed with a degree of modularity. This allowed for rapid adjustments as conditions in the deep ocean changed. It's easy to see how a system that could be quickly altered could provide a considerable advantage when working in the unpredictable underwater environment. Such adaptability was undoubtedly crucial for an operation of this scale and complexity.
Before any of these systems could be deployed, they underwent rigorous testing in simulated ocean conditions. This included high-pressure testing chambers that replicated the forces the equipment would face at extreme depths. One can imagine the pressure on the design and engineering teams to ensure their tools were not only robust but also reliable. Any failure at such depths would not only have been incredibly costly but also potentially very dangerous.
Ocean currents presented another considerable hurdle during the recovery. Their unpredictable nature meant that the grapple system had to be designed to handle forces that could tug and twist it during its operation. They undoubtedly drew on real-time data regarding currents to make decisions that ensured the grappling process was as efficient as possible. The level of detail required for these operations is quite astounding.
As the grappling process was underway, various monitoring systems kept a watchful eye on the condition of the remaining cable. The state of the cable on the seafloor was a critical consideration. The ability to constantly assess the cable's integrity provided valuable feedback to the recovery team, allowing them to adjust their methods as needed. This ongoing feedback process is a notable example of how the recovery engineers incorporated ongoing data and observations into their approach.
The innovation of the grappling system had broader implications beyond the immediate recovery operation. Its successful design and operation informed future subsea engineering. It would go on to influence underwater recovery techniques employed across a broad range of disciplines – including oil exploration, gas recovery, and the field of marine telecommunication. This type of cross-disciplinary fertilization is important in engineering.
The recovery of the 1865 cable was truly a collaborative effort. Mechanical engineers, marine biologists (to assess potential environmental impacts), and materials scientists all worked together on the project. The interdisciplinary collaboration was crucial in successfully completing this complex endeavor. The complexity of the operation is reflected in the numerous specialties that were involved.
Finally, the success of the recovery was bolstered by a data-driven approach. Engineers relied on simulations and analytics to guide their decision-making process. It's a testament to the foresight of the project's engineers that they utilized such modern data analysis and simulations, building off the lessons learned from prior cable projects. The approach underscores the importance of continuously adapting and refining engineering methodologies in the face of complex operational challenges.
The 1865 cable recovery, despite its challenges, was a resounding success that established important precedents for future underwater engineering endeavors. It's remarkable to consider that nearly 160 years after the project, we are still able to benefit from the ingenuity and creativity employed by engineers at the time.
Revolutionize structural engineering with AI-powered analysis and design. Transform blueprints into intelligent solutions in minutes. (Get started for free)
More Posts from aistructuralreview.com: