Engineering Marvel How New York City's 1904 Subway Construction Revolutionized Underground Transit Design
Engineering Marvel How New York City's 1904 Subway Construction Revolutionized Underground Transit Design - Cut and Cover Engineering Team Used 150000 Cubic Yards of Concrete in 1900 to Build First Line
The construction of New York City's inaugural subway line in 1900 relied heavily on the 'cut-and-cover' method, demanding a massive 150,000 cubic yards of concrete. This approach involved digging a trench, building the subway structure within it, and then sealing it back up, effectively restoring the street surface. It represented a bold step in urban planning, prioritizing the use of subterranean space within a rapidly expanding city. The scale of concrete usage itself signaled a shift in construction materials, highlighting a preference for durable and robust infrastructure. This approach to building subway lines, with its focus on efficiency and minimal surface disruption, went on to significantly impact future subway projects across the globe, establishing a model for urban transit design that emphasized underground infrastructure. The project, therefore, wasn't just about a new transportation system, but a demonstration of how engineering could adapt to overcome the unique challenges of urban environments.
The scale of the 1900s New York City subway construction, especially the first line, is truly striking. Using the cut-and-cover method, engineers poured an estimated 150,000 cubic yards of concrete—a mind-boggling quantity. It's a stark reminder of the monumental effort involved in carving out a transportation network beneath a bustling city. This approach, which essentially involved digging a massive trench, constructing the subway within it, and then sealing it back up, required intricate planning and coordination. One can only imagine the logistical challenges of managing such a massive concrete pour within the constraints of a built-up environment.
Interestingly, the utilization of this much concrete, while seemingly straightforward, reflects the engineering thinking of that era. Given the early 20th-century's construction methods, it's easy to see why such a material, known for its strength and durability, became the backbone of the project. Of course, today we might question the long-term impact on the urban environment, but at the time, concrete was viewed as a reliable, strong building block. This project became a significant example for the adoption of concrete on a grand scale in underground construction. It's a testament to the ambition of those early engineers, albeit we may now question if such an enormous use of concrete was the optimal choice for the environment.
We can appreciate that there are always trade-offs in engineering, and understanding the historical context is essential when evaluating past decisions. Even with the perspective of time and advancements in materials and engineering methods, it's important to acknowledge that this 1900s subway project was indeed a groundbreaking achievement that influenced subsequent urban planning and transportation engineering throughout the world.
Engineering Marvel How New York City's 1904 Subway Construction Revolutionized Underground Transit Design - Chief Engineer William Parsons Design of Compressed Air Tunnel Shields Protected Workers Below Ground
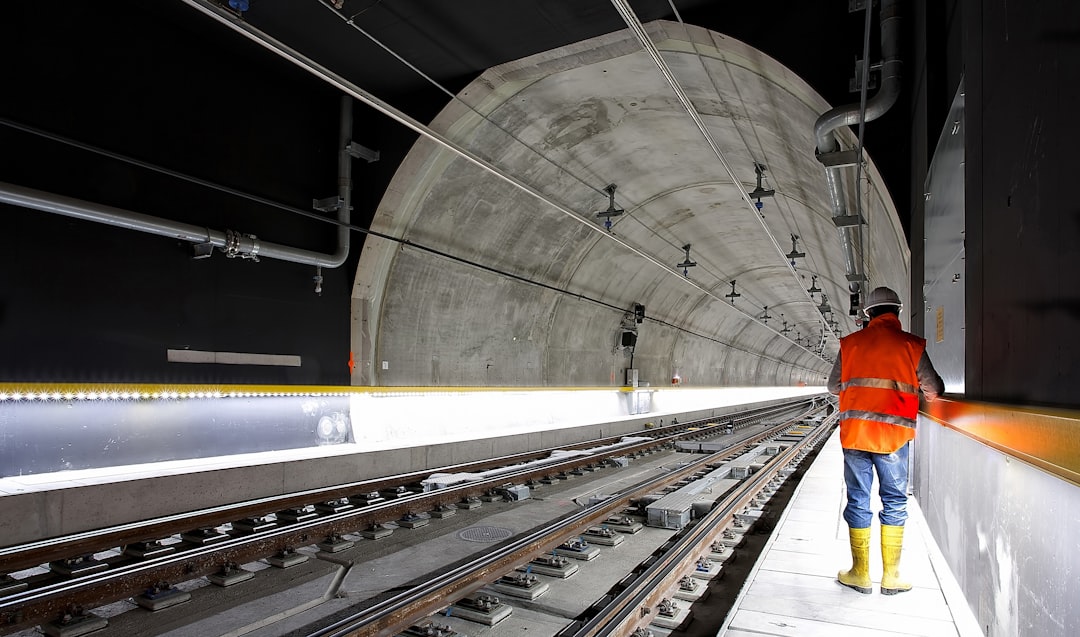
Chief Engineer William Parsons's design of compressed air tunnel shields was a crucial element in the successful construction of New York City's first subway line. These shields were a groundbreaking innovation, protecting workers from the inherent dangers of underground tunneling, such as cave-ins and the build-up of hazardous gases. The use of compressed air within the tunnels helped to maintain stability and create a safer working environment.
While these shields were a significant leap forward in engineering and safety, the project was still fraught with peril. The construction of the subway, while a remarkable achievement for urban development, unfortunately resulted in fatalities and injuries, demonstrating the inherent risks involved in such large-scale underground projects.
Parsons' innovative approach to tunnel construction went beyond the New York City subway project. His influence on civil engineering practices extended to other significant infrastructure projects, establishing him as a pioneer in this field. The successful completion and enduring legacy of the New York City subway system, particularly the 1904 opening of the first line, has served as a model for subsequent rapid transit projects globally, revolutionizing how cities approach underground transportation networks and showcasing how engineering could address the unique challenges of urban environments. This innovation proved that ambitious infrastructure projects could be successfully carried out in dense urban settings, influencing the development of urban planning and civil engineering across the world.
William Barclay Parsons, the Chief Engineer for New York's first subway system, was at the forefront of innovation when it came to underground construction. He designed compressed air tunnel shields, a crucial advancement that allowed workers to tunnel beneath the city's water table safely. The design incorporated compressed air to counteract the tremendous pressure exerted by the surrounding water and earth, effectively creating a safe working environment where the ground wouldn't collapse in on them. This was a huge leap forward from the earlier, more perilous, tunneling methods.
Parsons' use of compressed air wasn't just about speedier excavation. It dramatically reduced the risk for workers. Prior to his innovations, tunnel collapse was a significant hazard, but his designs helped mitigate this. However, this advanced technology also introduced a previously unseen challenge: "Caisson Disease", also known as decompression sickness. The rapid shifts in air pressure inside the tunnels led to some workers experiencing severe health problems, highlighting the unforeseen risks of innovative techniques. This serves as a potent reminder that even groundbreaking advancements can come with unexpected consequences.
The design of the shield itself was quite remarkable. Imagine a giant, metal teardrop – this was the fundamental shape of Parsons' invention. This unusual structure facilitated efficient tunnel boring while simultaneously offering resistance to the intense forces exerted by the surrounding environment. The project's success with the air shields became a pioneering example of pneumatic construction technology on a grand scale, influencing major projects across the globe.
Parsons' ingenious work involved sophisticated air pressure control systems, showcasing the intricacies of pneumatic engineering principles in a real-world application. The systems required a level of understanding of pneumatics that was quite sophisticated for the early 1900s. However, the advanced techniques came with a high price tag. Maintaining the air pressure and the associated infrastructure was financially demanding, pushing the project’s budget.
Beyond the technological aspect, Parsons’ design considered the urban environment itself. By minimizing surface disruption, his methods demonstrated an early example of how engineering could work within an existing city. The subway project wasn't just about digging a hole; it was about seamlessly integrating a new transportation system into the fabric of the city, a concept that is remarkably relevant today.
The impact of Parsons’ tunnel shield technology extends far beyond the New York City subway. His innovations laid the groundwork for many modern tunneling techniques, demonstrating how a single project could transform entire engineering disciplines. While acknowledging that there are always tradeoffs in engineering, it’s evident that Parsons' contribution to urban transit was transformative and his influence can be felt even today in subway systems and other construction projects around the world.
Engineering Marvel How New York City's 1904 Subway Construction Revolutionized Underground Transit Design - Manhattan Schist Rock Formation Led to Unique Excavation Methods at 125th Street Station
The 125th Street Station's construction presented a unique challenge due to the presence of Manhattan Schist, a remarkably strong and ancient rock formation underlying the city. This rock, formed approximately 450 million years ago during the Pangea supercontinent era, presented a tough material to work with. Its inherent strength and durability, while beneficial for supporting the weight of buildings and tunnels, necessitated innovative excavation methods. Engineers had to carefully consider the schist's properties during the station's construction, ensuring that the subway structure would be both stable and integrated with the existing geological landscape. This experience showcases how the interplay between geological constraints and engineering solutions significantly impacted the development of New York City's subway system and ultimately shaped the design of urban infrastructure. The choices made during the construction of the 125th Street station serve as a reminder of how geological features play a vital role in influencing the engineering of modern cities, especially when building extensive underground structures. These early engineering challenges helped pave the way for how we design and construct urban transportation today.
Manhattan Island's bedrock is predominantly composed of Manhattan Schist, a metamorphic rock formed around 450 million years ago when all the continents were part of the supercontinent Pangea. This rock, a mixture of biotite, gneiss, and schist, is remarkably strong and durable, thanks to its high quartz and feldspar content. This strength, while beneficial for supporting the weight of buildings and infrastructure, presented unique challenges for engineers working on projects like the 125th Street subway station.
The extreme hardness of Manhattan Schist meant that traditional excavation methods simply wouldn't suffice. Engineers had to devise more powerful approaches, relying on techniques like drill-and-blast or heavy-duty mechanical excavators to break through the rock. At the 125th Street station, they also turned to hydraulic power to provide the necessary force to remove large chunks of schist, showing how engineering advanced beyond earlier, more manual methods.
Interestingly, the depth of excavation required for the subway lines varied depending on the specific characteristics of the schist layer encountered. Variations in hardness and weathering required adjustments to the planned construction process to mitigate potential problems like instability or water infiltration.
Despite the intense excavation work, one unexpected benefit of the schist was that its density naturally muffled the noise of construction. The rock acted as an acoustic barrier, reducing sound transmission to the surface, which was a critical aspect of the project given the heavily populated urban environment.
Prior to the excavation, extensive geological surveys were essential to understand the rock’s characteristics at different depths. This geological data, along with insights into the rock’s composition, provided engineers with the information needed to plan for the most effective and safe excavation approaches.
The strength of the Manhattan Schist also affected the design of the subway tunnels themselves. Because the rock is so strong, engineers were able to build tunnels with larger vertical and horizontal clearances than would be possible in a softer substrate. This feature of the schist allowed for optimized tunnel sizes and contributed to the long-term ability to potentially expand and adapt to future needs.
However, this strength also presented other design challenges. The schist's heat retention properties create considerable temperature fluctuations within the tunnels, demanding careful design and implementation of ventilation systems to ensure the tunnels remain functional and passenger-friendly.
Beyond the engineering feats, the construction of the first subway lines through Manhattan Schist is a crucial piece of New York City's history. It demonstrated how engineers were able to take advantage of the city’s geology to create a functional and efficient underground transportation system. This approach, transforming New York into a city that efficiently used its subterranean space, has influenced urban planning and underground construction across the globe.
Finally, the inherent stability of the Manhattan Schist post-excavation provided a natural support system, a distinct advantage compared to projects where excavation could potentially lead to ongoing instability. Despite this, continuous monitoring of the underlying geological structure remains a crucial part of ensuring the long-term safety and structural integrity of the subway network. While we can celebrate these historical engineering feats, it is vital to realize that the city’s infrastructure is constantly evolving, and careful study and attention to such fundamental aspects as geological stability remains vital.
Engineering Marvel How New York City's 1904 Subway Construction Revolutionized Underground Transit Design - Beach Pneumatic Transit Experimental Tunnel in 1870 Provided Critical Lessons for 1904 Design
Alfred Ely Beach's Beach Pneumatic Transit, launched in 1870, represented New York City's initial attempt at a subterranean public transit system. This experimental project, utilizing compressed air to move a single-car train along a short stretch of Broadway, provided valuable, albeit unexpected, lessons for the design of the 1904 subway. While ultimately deemed impractical for broader use and discontinued just a few years later, it demonstrated the possibilities and limitations of air-powered transit and introduced some design challenges that later engineers would address.
The Beach Pneumatic Transit, though short-lived, was a critical stepping stone in the development of New York's subway. Its innovative approach, while not fully realized, showcased both the allure and the pitfalls of pioneering technologies in transit. The project's failure due to limited practicality helped shape a deeper understanding of the requirements for creating a truly functional and scalable underground transit network. The 1904 subway builders could learn from Beach's endeavors, leveraging some of his insights while avoiding his missteps, and ultimately contributing to a far more enduring system that became a worldwide model for rapid transit engineering. The Beach Pneumatic Transit stands as a reminder of the trial-and-error nature of innovation in engineering, particularly in developing complex systems in challenging urban environments. It's a testament to how early explorations and failures can lead to the development of remarkable, long-lasting achievements.
Alfred Ely Beach's Beach Pneumatic Transit, a pioneering attempt at underground transit in New York City, emerged in 1870. It was a bold concept, utilizing compressed air to propel a single-car shuttle through a short tunnel beneath Broadway. While a fascinating experiment, its brief lifespan ended in 1873 due to a combination of financial hurdles and inherent limitations in its design. Despite its ultimate failure, the Beach Pneumatic Transit provided invaluable lessons for the engineers who tackled the vastly more successful 1904 subway project.
The most notable outcome of the Beach Pneumatic Transit experiment was the increased understanding of the crucial role of ventilation within underground spaces. The initial design struggled to maintain adequate air quality, highlighting the necessity of robust ventilation systems for passenger comfort and safety – a lesson integrated into the 1904 subway plans. Furthermore, the use of compressed air in the Beach Pneumatic Transit sparked further exploration of pneumatic technologies. These explorations culminated in Chief Engineer William Parsons' ingenious application of compressed-air tunnel shields for the 1904 subway, allowing for safer and faster excavation.
Beyond the technical aspects, the Beach Pneumatic Transit exposed the significance of comprehensive geological surveys and pre-construction research. The 1870s endeavor encountered unexpected challenges that might have been mitigated with more thorough initial research. This emphasis on geological knowledge became a critical factor in the planning and execution of the 1904 subway. Likewise, the Beach Pneumatic Transit highlighted the need for improved worker safety measures within underground environments. The experience revealed gaps in existing materials and methods, prompting the development of stronger and more robust tunnel construction techniques, subsequently adopted in the 1904 project.
Moreover, the Beach Pneumatic Transit illustrated the complex interplay between ambitious engineering projects and the wider urban context. It emphasized that the success of such ventures hinges on public perception and engagement. It seems clear, from the failed venture, that engineers need to fully consider the community and political landscape for major infrastructure undertakings. Similarly, the inherent design flaws and operational challenges that the Beach Pneumatic Transit revealed – such as difficulties in maintaining constant air pressure and its overall structural limitations – played a vital role in shaping the more resilient and successful design of the 1904 subway.
While the Beach Pneumatic Transit ultimately failed as a long-term transportation solution, its legacy as a catalyst for innovation in urban transit design cannot be understated. The fundamental principles learned during this experimental endeavor formed the building blocks of future subway designs, particularly those realized in 1904. In this sense, the Beach Pneumatic Transit's story illustrates a crucial concept in engineering – that even failures can be remarkably instructive, guiding future innovation and advancing the field. The insights derived from its brief but ambitious life became integral to the success of later, more sustainable underground transit projects, cementing its unique role in the development of urban transportation in New York and across the globe.
Engineering Marvel How New York City's 1904 Subway Construction Revolutionized Underground Transit Design - Interborough Rapid Transit Company Built 350 Steel Support Columns Under Park Avenue in 1902
In 1902, the Interborough Rapid Transit Company (IRT) embarked on a significant undertaking: the construction of 350 steel support columns beneath Park Avenue. This served as a crucial precursor to New York City's first subway line, demonstrating a commitment to robust infrastructure and setting the stage for innovative engineering techniques in underground transit design. The project, involving a substantial amount of steel—over 74,000 tons—highlighted a shift towards a more durable approach to urban infrastructure construction. The IRT's decision to utilize steel columns beneath Park Avenue reflected a determination to overcome the challenges of building a subway system within a densely populated city. The success of this endeavor marked a turning point, not just for the IRT's subway project, but also for future urban infrastructure projects globally, showcasing the potential of bold engineering solutions. It underscored that complex underground projects could be successfully integrated within urban environments.
The Interborough Rapid Transit Company's (IRT) subway project, launched in 1902, required the construction of 350 steel support columns beneath Park Avenue. This decision was driven by the need to manage the immense weight of the infrastructure above the subway and underscores the complex engineering challenges faced when building in urban environments, where space is at a premium and weight distribution is critical. It marked a shift from traditional, possibly more rudimentary, masonry support systems to a more modern approach employing steel, highlighting the adoption of new materials in early 20th-century construction.
Each of these 350 columns was carefully rooted in the bedrock below, a testament to the sophisticated understanding of foundational engineering at the time. They were designed to withstand the dynamic forces generated by the subway trains and the city's infrastructure above, ensuring the stability and longevity of the project. Standing over 100 feet tall, the fabrication and installation of these columns necessitated exceptional precision. Any deviation could have severely compromised the structural integrity of the entire subway network and the surrounding buildings, emphasizing the importance of exacting standards in such a complex undertaking.
It's intriguing that steel was selected over concrete for the support columns. This choice reflects a prevailing engineering trend in the early 1900s where steel was becoming recognized as a safer and more resilient material for supporting significant structural loads compared to older materials like brick or stone. The sheer quantity of steel utilized in the project likely contributed to advancements in steel production techniques. Manufacturers were pushed to develop higher-quality steel that could endure the constant, sometimes intense, stresses generated by subway operation.
The location of these columns allowed for deeper excavation while minimizing disruption to surface infrastructure. This was a pioneering concept that foreshadowed later urban tunneling projects and emphasized the importance of seamlessly integrating new infrastructure into existing urban environments. It's likely that the columns were designed to accommodate some movement, acknowledging the impact of dynamic loads. Structures like these, subjected to vibration from train travel, earthquakes, and other environmental factors, require a level of flexibility to avoid failure.
Furthermore, sophisticated engineering calculations were used to predict load distribution across the network. This was forward-thinking for the time, indicating a clear desire to build a robust system capable of handling increasing passenger traffic in the future. The very existence of these columns under Park Avenue exemplifies a paradox that continues to influence urban engineering: while supporting vital infrastructure, they also symbolize the inherent challenges of working within the constraints of dense urban settings, a reality that remains a challenge today.
Engineering Marvel How New York City's 1904 Subway Construction Revolutionized Underground Transit Design - Electric Power Innovation at 59th Street Powerhouse Enabled Safe Underground Operation
The 59th Street Powerhouse, built in 1904, was crucial for powering New York City's first subway, the Interborough Rapid Transit (IRT) system. Its design, a blend of innovative electrical engineering and a beautiful Beaux-Arts style, was a symbol of the era's technological progress. The shift towards electric power, especially using newly developed electric motors, was key to making the subway a feasible project. This represented a notable leap in engineering, allowing for the creation of a safe and efficient underground transit system.
Despite its historical significance, the powerhouse has faced calls for demolition, which raises important questions about the preservation of vital historical structures tied to engineering achievements. It remains a tangible reminder of how engineering innovation revolutionized transportation in a rapidly growing metropolis, emphasizing the crucial role of electricity in transforming urban infrastructure. It serves as a testament to the ability of humans to utilize technology to build systems that meet the demands of a city's growth and serve a crucial public purpose.
The 59th Street Powerhouse, inaugurated in 1904, was a pioneering facility built to power New York City's first subway system, the Interborough Rapid Transit (IRT). Its location, nestled between West 58th and 59th Streets, Eleventh and Twelfth Avenues, and alongside the North River, was strategically chosen. The building itself is a fascinating blend of early 20th-century engineering and Beaux-Arts architectural style, designed by the renowned architect Stanford White. The design was informed by a careful study of existing large powerhouses, reflecting the IRT Company engineers' desire to create a robust and efficient facility. It's no surprise the facility became known as the "Temple of Power", highlighting its role as a critical element in the electric revolution of that era.
The rise of electric motors in the mid-1890s made the subway a feasible concept. This technological advancement paved the way for the subway's construction, which commenced in 1900 and concluded in 1904. The powerhouse's electrical machinery contract, signed in October 1901, was awarded to Westinghouse Electric and Manufacturing Company by John B. McDonald, the main contractor for the subway line. The powerhouse stands as one of the oldest surviving power stations in the city, though it's faced periods of vulnerability and threatened demolition in recent years, which suggests that its historic value has not always been fully recognized.
Its construction was crucial to the evolution of underground transit design. It's often described as an engineering marvel, demonstrating the advancements of the era. Beyond its technical prowess, it was a crucial part of a broader transformation of urban transportation and a landmark for the adoption of electric power for public transit, emphasizing how the city was adopting new infrastructure within its already developed, and very constrained, urban environment.
The powerhouse embraced innovative electrical distribution methods, specifically the large-scale adoption of alternating current (AC) technology. It was a crucial development as AC electricity could be transmitted efficiently over long distances, crucial for the sprawling subway network. Interestingly, the design emphasized safety through various electrical protections, like grounding and circuit breakers, particularly relevant given the underground location and the close proximity of the rails to the public.
Engineers opted for a dual energy system. Alongside the electric generators, hydraulic systems were integrated into the powerhouse, possibly leveraging the adjacent river as a source of energy. The sheer scale of this hybrid system is evident in the fact that the cooling system alone was capable of processing up to 50,000 gallons of water per minute, a testament to the scale and ambition of the project. It seems clear, given its design, that the facility was meant to handle large variations in demand, which would have occurred based on the ebb and flow of subway traffic, and the need for a cooling system was likely driven by the significant heat output of the generation equipment.
The design process didn’t overlook the urban context. It was built in a way to address the natural heat from the earth's core in the Manhattan area. It also supported not just the subway but also nearby commercial and residential buildings. That shows some foresight into building a multi-functional utility system, reflecting early ideas of infrastructure integration. The facility incorporated vertical shafts for electrical distribution, allowing for easier maintenance and reducing surface disturbance. These kinds of features suggest the engineers were thinking beyond just getting the subway operational, but developing a sustainable and safe system.
In addition to power generation and distribution, the 59th Street Powerhouse incorporated communication signals. This is interesting as it was still the dawn of electrical communications. This integration is notable because it shows that the facility was designed to operate in a comprehensive manner. The power and communications systems weren't viewed as isolated pieces of infrastructure. The facility also implemented load-balancing methods, demonstrating their forward thinking in ensuring a reliable power supply for the subway.
The 59th Street Powerhouse is a reminder of the vital role that innovation played in the birth of modern subway systems, and urban transport as a whole. Its design and operation contributed to standards for future urban electricity distribution, establishing it as a touchstone for the development of safe, efficient, and reliable power infrastructure in other major cities around the globe. Its story, therefore, is not merely about a power plant, but about the innovative engineering that underpinned the modernization of urban life.
More Posts from aistructuralreview.com: