Steel Beam Span Rule of Thumb Practical Guidelines for Structural Engineers in 2024
Steel Beam Span Rule of Thumb Practical Guidelines for Structural Engineers in 2024 - Span-to-Depth Ratios for Different Beam Types in 2024
Span-to-depth ratios continue to be a key consideration in beam design. Current practice suggests a 1:5 ratio for roof beams, while floor beams generally fall closer to 1:6. Composite beams often settle somewhere in between at roughly 1:5.5. For steel beams, a handy rule of thumb persists: convert the span from feet to inches and divide by 20 to estimate the required depth. However, this is just a starting point and needs to be verified for the specific loading conditions.
Coupling beams present a unique challenge, with depth ratios ideally kept between 0.80 and 0.95 of the composite beam's total depth. This constraint emphasizes the necessity of designing custom reinforcement for these elements to address varying span-to-depth ratios. Ultimately, finding the optimal balance between these ratios and meeting load demands requires careful consideration of project specifics and relevant standards, as well as awareness of the evolving best practices. There remains some debate and revision of past thinking in this area which suggests continued refinement may be in store.
Delving deeper into specific beam types, we find that span-to-depth ratios vary considerably. For instance, roof and floor joists often utilize ratios of 1:5 and 1:6, respectively. It's interesting to note that composite beams tend to fall somewhere in between, around 1:5.5. This suggests a possible optimization strategy where composite construction can potentially bridge the gap between the needs of roof and floor systems.
A quick rule of thumb for steel beams involves converting the span from feet to inches and then dividing by 20. While useful, we should acknowledge that it is a simplification and might not be universally accurate. For example, a 30-foot span would suggest a beam depth of 18 inches. The appropriateness of this rule would vary greatly depending on loading and other factors, but it can be a good starting point.
The depth-to-span ratios also vary based on application. For roof and floor joists, the acceptable depth is within 0.5 to 0.6 times the span, and 0.55 to 0.6 times for floor joists, respectively. There seems to be a slight discrepancy in acceptable depth depending on the application. It would be interesting to explore whether this difference is truly necessary or a conservative approach based on typical loading.
When it comes to coupling beams, which are often part of composite structures, the span-to-depth ratio needs careful consideration. It is suggested to be limited to 0.80 to 0.95 times the overall depth of the beam. This restriction seems important to ensure a good bond and prevent potential shear failure. The design considerations in such a system highlight the critical need for a nuanced understanding of the interdependency of components.
Minimum shear reinforcement is mandated regardless of the span-to-depth ratio, emphasizing the need for robust shear design. This is a general safety requirement, ensuring that certain loads are accounted for in all cases. Interestingly, minimum embedment length in coupling beams seems to be linked to the span-to-depth ratio, underscoring that reinforcement design needs to be tailored to each specific scenario.
As an example, for a 35-foot steel beam span, a W16 or W18 profile might be suitable, again, contingent on the exact loading and spacing. This highlights the fact that multiple beam sections can suffice depending on load requirements, demonstrating the flexibility but also the complexity inherent in steel design.
A commonly accepted span-to-depth ratio for uniformly loaded steel beams sits around 18 to 20. It appears that there is a preference toward this ratio in many design situations, indicating its possible wider suitability in standard cases. However, this is just a general observation, and a closer look into code provisions and the particular design constraints is still critical.
The potential depth for steel beams, particularly when considering things like spacing and loading, seems to vary depending on the span, with depths being specified for spans between 20 and 28 feet. It appears there are some typical span ranges and corresponding beam depths that are considered standard.
Regarding reinforced concrete continuous beams and slabs, the span-to-depth ratios are proposed based on meeting ductility requirements. This highlights a shift from merely fulfilling deflection limits, and the focus on ductility underscores the importance of the structural behavior and potential failure modes.
Steel Beam Span Rule of Thumb Practical Guidelines for Structural Engineers in 2024 - Calculating Nominal Beam Depth for Specific Live Loads
Determining the appropriate nominal depth for steel beams, especially when dealing with varying live loads, is fundamental to achieving both structural soundness and material optimization. For example, a W36 beam spanning 35 feet and supporting a live load of 100 psf might require a depth between 16 and 18 inches. A commonly used rule of thumb involves estimating the beam depth by dividing the span (in feet) by 20, but this is just a starting point. Engineers must carefully verify these estimations against the actual anticipated loads to ensure adequate strength. The optimal depth-to-length ratio also changes depending on the beam's function, with recommendations ranging from 0.5 for roof beams to 0.6 for floor beams. These ratios highlight the need to consider how different live loads and material properties can impact beam design. Moreover, continually evolving standards and regulations make it critical for engineers to utilize precise calculations to guarantee safety and structural stability while managing live load requirements. This complex process demands a thorough understanding of both structural principles and current best practices to ensure that designed beams can reliably perform as intended.
1. The determination of a beam's nominal depth for specific live loads can be significantly affected by the anticipated duration of those loads. Shorter-duration loads might permit the use of shallower beams, which in turn can influence the overall structural design considerably. It would be interesting to explore how this interaction between load duration and depth is currently being incorporated in design practice.
2. When designing beams for live loads, dynamic factors like accidental impacts or vibrations are often overlooked. This simplification might lead to underestimation of the required beam depth, as these additional forces could necessitate stronger reinforcement. It's concerning that these dynamic aspects are often not explicitly considered and might be a source of potential design inadequacies.
3. Building codes classify live loads across a wide spectrum, from typical office environments to heavily trafficked public areas. The sensitivity of beam depth requirements to these usage variations highlights how even minor alterations in anticipated activity can substantially change the structural demands on a beam. Perhaps a more granular classification of live loads could be beneficial in improving design accuracy and efficiency.
4. The shear strength of a beam can have a substantial impact on nominal depth calculations. Increased shear forces in certain regions of a beam might necessitate deeper sections to fulfill safety requirements, which can complicate the design process. It's important to better understand how shear strength and depth interplay during the design phase.
5. While common guidelines suggest using a nominal span-to-depth ratio, localized demand factors, such as point loads or concentrated forces, can make the necessary beam depth deviate considerably from typical expectations. It seems as though these local load concentrations are a factor that can easily lead to design oversights, especially in preliminary design phases.
6. Recent developments in finite element analysis (FEA) software have enabled more accurate assessments of how actual load conditions affect nominal beam depth. This advanced technology allows for a more precise optimization of design parameters compared to traditional rules of thumb. However, the accessibility and adoption of these tools might vary across engineering firms, creating a potential for disparity in design quality.
7. The mechanical properties of steel, surprisingly, are sensitive to temperature fluctuations. This factor necessitates adjustments in nominal beam depth for structures located in areas with extreme climates. These changes in steel's behavior can impact load capacities and demand changes in design practices. Perhaps future standards should incorporate more explicit temperature-dependent factors for steel in design guidelines.
8. Beam depth calculations should also factor in lateral-torsional stability. Variations in the beam's geometry and load distribution can lead to unexpected buckling behavior, which might necessitate using a deeper beam than originally planned. It's critical to understand the intricacies of this phenomenon and its influence on design parameters.
9. Sometimes, engineers leverage empirical data from similar projects to adjust the nominal beam depth for specific live loads. This practice demonstrates how shared knowledge and past experiences can refine design approaches beyond a strict adherence to code provisions. However, caution is warranted when relying solely on past examples as load and design considerations may vary significantly between projects.
10. The continuous evolution of composite construction techniques implies that nominal beam depth frequently requires meticulous adjustments based on the interplay between materials, such as steel and concrete. This highlights a dynamic environment for structural engineering design, where constant learning and adaptability are paramount. It would be useful to conduct further research into the best practices for material pairings within composite beams and how to translate these learnings into design guidelines.
Steel Beam Span Rule of Thumb Practical Guidelines for Structural Engineers in 2024 - Impact of Span-to-Depth Ratio on Preliminary Design
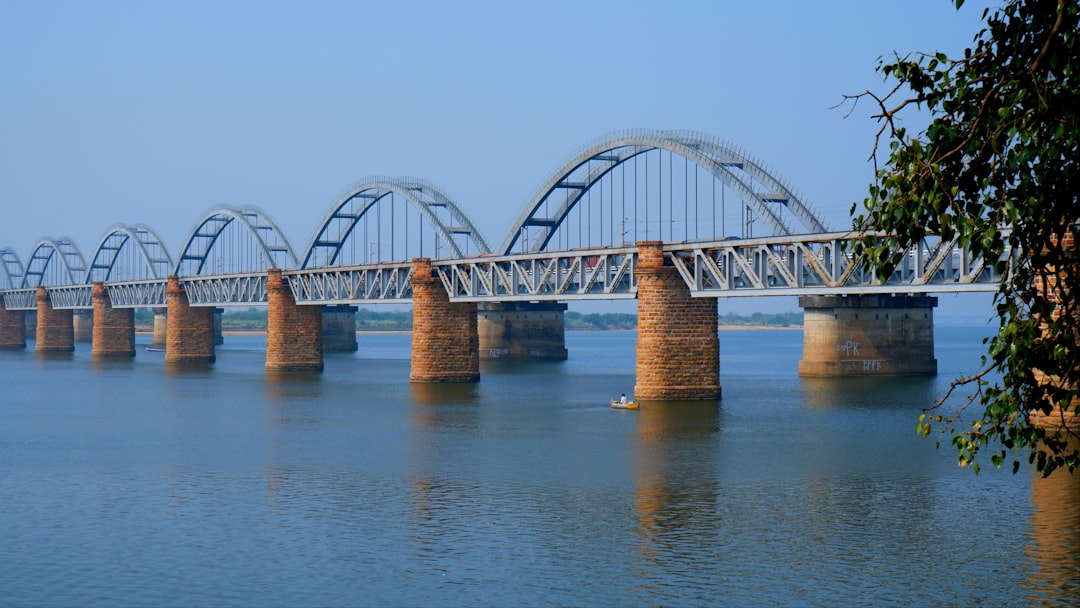
The span-to-depth ratio plays a pivotal role in the initial design stages of steel beams, affecting both their strength and how much they bend under load. Finding the right balance between the beam's length (span) and its height (depth) is crucial for both structural integrity and material efficiency. Generally, a span-to-depth ratio between 12:1 and 20:1 is considered reasonable, though this range shifts depending on the specific beam application (like roof or floor beams) and how it will be loaded. It's important to remember that these preliminary estimations need further refinement. Factors like the steel's properties and adherence to current design standards need to be incorporated to achieve a truly optimized design. The ongoing changes in design practices highlight the need for engineers to stay updated and refine their approaches to achieve optimal structural performance. Failing to consider this critical relationship can have significant consequences on the final beam design, so it's something that needs careful consideration from the start.
1. The span-to-depth ratio isn't just about structural integrity; it also impacts practical considerations like available headroom and overall ceiling height. A larger beam depth can eat into valuable vertical space, leading to potential conflicts with architectural and interior design goals.
2. It's surprising how often the aesthetic impact of beam depth is overlooked. In some projects, having very deep beams can disrupt the visual balance of a space, creating a point of contention between structural and architectural aims. Clients and architects might prioritize a more harmonious look, potentially impacting the initial structural choices.
3. While a smaller span-to-depth ratio usually gives you a stiffer beam, it also increases the beam's own weight. This might require stronger or more supports, potentially undoing the initial benefits. It's a balance between stiffness and the implications of added weight on the overall system.
4. Looking at real-world scenarios shows that beams with a 1:6 span-to-depth ratio might face unexpected bending stresses when dealing with live loads that aren't evenly distributed. This underscores the importance of carefully assessing how loads are applied and distributed across the structure.
5. Interestingly, some design codes haven't kept up with the need for refined span-to-depth optimization beyond the basic recommended ranges. This puts more emphasis on engineers creatively leveraging empirical data and practical experience rather than relying strictly on established design guidelines.
6. Recent studies suggest that making small, calculated adjustments to the span-to-depth ratio based on the specific site and loads can result in using less material. This points to the potential for a more tailored approach to design that can optimize resource use.
7. Some researchers believe that larger span-to-depth ratios could actually lessen the impact of dynamic loads in certain situations. This goes against the typical assumption of always minimizing the ratio, highlighting the need for a more nuanced understanding of how design choices affect beam behavior in specific applications.
8. Smaller span-to-depth ratios can create hurdles during construction, particularly in places with limited space or access. Maneuvering equipment and materials around these beams can become a problem. This highlights how important practical construction challenges are for the structural design phase.
9. The rapid advancement in composite beam technologies has sparked a reevaluation of how we think about span-to-depth ratios. Using new materials can potentially lead to design solutions that are significantly different from those achievable with traditional steel beams.
10. A deeper look into design and construction practices reveals that even small changes in how a building is built can have a meaningful effect on how the beam is expected to behave. This is particularly true for those ratios that were previously seen as more fixed values. It underlines a dynamic relationship between design decisions and actual construction processes.
Steel Beam Span Rule of Thumb Practical Guidelines for Structural Engineers in 2024 - Common Steel Beam Types and Their Applications
Steel beams are available in a range of shapes and sizes, each tailored for specific construction applications. Commonly encountered are I-beams (also known as W-beams) and H-beams, favored for their robust nature in supporting floors, roofs, and other elements in various building types. These beams excel in situations demanding high strength and stability. In contrast, angle beams, with their L-shaped profile, are typically employed for smaller-scale applications such as brackets or simple framing members. Their lightweight design and favorable strength-to-weight ratio are key advantages. Architects and engineers must carefully consider the unique properties of each beam type during design, as a mismatch in beam selection can compromise the structural integrity of a project. The right beam choice also becomes a factor in meeting current building codes. As the field of construction continuously evolves, structural engineers must stay current on the latest guidelines and design advancements to ensure safety and efficacy within projects. Staying up-to-date and applying the most appropriate beam types remains critical for optimized structural outcomes.
1. The form of a steel beam, especially the wide-flange type (W-beam), plays a big part in how well it works. The orientation of the flange—whether it's up and down or side to side—affects how well it withstands bending and twisting, depending on how the loads are applied. It's fascinating how a simple change in orientation can have such a big impact on structural performance.
2. When it comes to reducing how much a beam bends under a load, the depth of a steel beam can be more important than how strong the steel itself is. Making a beam deeper can significantly cut down on deflection, which means you might be able to use less material while still getting good performance. This illustrates the complex trade-offs engineers face between depth, weight, and overall strength when designing structures.
3. Composite beams, which combine steel and concrete, are becoming more common as a way to overcome limitations on how long a span can be. They allow for longer spans with shallower beams, which makes them more efficient overall. This interesting combination can lead to cheaper construction and stronger load-bearing capacity, which makes them a very promising option compared to older methods.
4. In earthquake-prone areas, choosing the right steel beam involves more than just looking at span-to-depth ratios. Engineers need to consider a beam's ductility—its ability to bend and deform without breaking—since this is crucial for making sure structures can withstand earthquake forces without collapsing. This emphasizes the importance of understanding the behavior of materials under extreme conditions.
5. It's easy to make mistakes when designing if you assume all beams respond the same way to loads. In reality, concentrated loads can have very localized effects, which requires engineers to adapt their designs in unique ways. If not handled carefully, these effects can significantly alter the expected behavior of the beam and potentially lead to safety issues. It highlights the need for a very detailed understanding of load distribution.
6. Understanding the "section modulus" is crucial for beam design because it affects how strength is calculated when beams are bending. Having a bigger section modulus helps reduce stress concentrations, which is key when choosing the right beam profile for a particular application. It influences not only structural safety, but also design choices related to aesthetics.
7. It's intriguing how the way a steel beam is attached to the rest of the structure can have a huge impact on its strength. Poorly designed connections can create stress concentrations, which can limit the beam's overall performance. It shows that even small parts of a design need to be done meticulously to ensure the structure performs as expected.
8. Advanced simulation tools like nonlinear finite element analysis allow for more detailed predictions of beam behavior in complex situations. This has dramatically changed how engineers design because it lets them consider realistic variables that traditional methods might miss. This shift towards advanced analysis suggests an exciting evolution in structural engineering.
9. Research suggests that deeper beams can better resist a phenomenon called lateral-torsional buckling, especially in longer spans. This is crucial for ensuring that beams remain stable under load. It shows that simple design proportions can greatly affect the choice of material and the ultimate performance of a beam.
10. The use of beams with varying depth along their length—meaning the beam's depth changes throughout the span—is becoming more common. This approach lets engineers tailor the beam's response to specific loading conditions, which can improve material efficiency and potentially reduce project costs. This innovative method potentially offers more effective ways to build structures.
Steel Beam Span Rule of Thumb Practical Guidelines for Structural Engineers in 2024 - Balancing Deflection Criteria with Span-to-Depth Guidelines
Structural engineers face the ongoing challenge of balancing deflection limitations with appropriate beam dimensions, specifically the relationship between span and depth. Deflection control is critical for maintaining serviceability, preventing issues like excessive floor movement or sagging under load. While a general span-to-depth ratio of 1:12 might be a starting point, engineers must consider the specific application. Factors such as anticipated loads, the type of beam being used, and the overall intended purpose of the structure can significantly affect the acceptable span-to-depth ratio. Failure to carefully consider these factors can lead to beams that are either too flexible or unnecessarily large. Furthermore, recognizing how actual load conditions differ from simplified assumptions allows for more efficient material usage and potentially improved structural performance. This complex interplay requires ongoing adjustments as industry standards and best practices continue to evolve, pushing structural engineers to adopt adaptive design approaches for optimized outcomes.
1. The interplay between deflection limits and span-to-depth recommendations reveals an interesting trade-off: striving for minimal deflection frequently results in using more material, potentially increasing costs and structural weight. This necessitates a careful assessment of a project's overall goals to strike a balance.
2. An often-overlooked aspect is the effect of beam depth on a space's acoustics; deeper beams can help reduce sound transmission, offering a beneficial side effect in certain scenarios. It's intriguing how this aspect can be integrated into design choices.
3. When examining beam design guidelines, it's notable that allowable deflection is usually more restrictive for floors than roofs, reflecting the distinct usage and performance requirements for each. This difference can be surprising to those new to structural design.
4. Mathematical models sometimes underestimate the impact of live load fluctuations; employing conservative estimations can lead to excessively deep beams, potentially overlooking opportunities to save costs and optimize material usage.
5. Engineers encounter a unique challenge as span-to-depth guidelines evolve—while traditional recommendations often apply, innovative materials and construction methods sometimes deviate from established practices, highlighting the importance of continuous professional development within the field.
6. There's a crucial intersection between building codes and engineering judgment; certain projects might not align perfectly with standard criteria, emphasizing the dynamic relationship between regulatory requirements and novel design approaches.
7. Interestingly, the increasing use of advanced materials like high-strength steel can facilitate shallower depth-to-span ratios than previously thought possible, demonstrating the evolving nature of engineering practice as technology advances.
8. The concept of "camber," or the intentional slight upward curve built into a beam, significantly influences deflection control but can also create unexpected visual impacts in finished structures, influencing how architects and engineers approach aesthetics.
9. The dynamic behavior of beams subjected to oscillating loads is often overlooked, yet it can strongly inform decisions about depth and material selection, particularly in situations where vibrations are common, such as bridges or large auditorium spaces.
10. The span-to-depth ratio's influence on local buckling within beams can be surprising to engineers, highlighting the intricate behavior of materials that must be carefully considered during design, especially for longer spans supporting significant loads.
More Posts from aistructuralreview.com: