Structural Analysis of Woodstock's Middle Covered Bridge A Modern Take on Historic Design
Structural Analysis of Woodstock's Middle Covered Bridge A Modern Take on Historic Design - Design Elements Blending 19th and 21st Century Engineering
The design of bridges, particularly those with historical significance like Woodstock's Middle Covered Bridge, reveals a compelling interplay between the engineering traditions of the 19th and 21st centuries. The 19th century witnessed remarkable progress in bridge construction, particularly with wooden truss designs that enhanced strength and span, laying the foundation for later innovations. However, contemporary structural engineering has moved forward, leveraging advanced materials and methodologies, like those seen in the innovative cable-stayed systems of bridges like the Millau Viaduct. The ability to incorporate modern engineering know-how while respecting historical design principles presents a complex but crucial challenge. Finding the balance between the old and the new is essential when modernizing historical structures, as seen in the need to carefully integrate modern techniques and materials into the repair or restoration of the Woodstock bridge. This effort underscores a fascinating intersection of historical legacy and contemporary technological capabilities, fostering a deeper comprehension of how structures are designed and built within the ever-evolving landscape of engineering.
The historical development of bridge engineering, particularly in the 19th century, saw a reliance on timber and truss systems, often tailored to locally available resources. This stands in contrast to the 21st century's focus on standardized materials and methods, revealing a shift in engineering priorities. Furthermore, the 19th century's reliance on empirical observations and traditional techniques differed from modern engineering's emphasis on computational modeling and quantitative analysis. This shift provides interesting questions about how the evolution of knowledge and technology influences design and how we value past craftsmanship versus the promise of data-driven decision-making.
The 21st century has witnessed remarkable advancements in materials science, enabling a new palette for structural design. High-strength steel and reinforced concrete now play central roles in bridge construction, delivering solutions that were not readily available to the engineers of the past. We can achieve a greater blending of aesthetics and structural integrity with modern materials, moving beyond the heavier, more simplistic timber truss systems. However, this change also raises questions about the loss of traditional techniques, particularly the skills associated with woodworking and intricate joinery. Modern digital fabrication methods may provide options for replicating historic forms with precision but potentially at the cost of developing a future generation of skilled craftspeople.
Modern design considerations have evolved considerably. Factors such as safety and load capacity are addressed far differently than in the 19th century. Bridge designs today face significantly greater loading considerations, particularly in high-traffic areas. The introduction of codes and regulations designed to ensure safety has had a significant impact on design, moving away from simpler solutions with lower load ratings. Additionally, new technologies like BIM offer valuable visualization capabilities, enabling engineers to fully understand and monitor the bridge throughout its lifecycle. This level of comprehensive insight is a departure from the empirical methods of past engineers who relied on experience and assumptions when dealing with a bridge's unseen interior structure and long-term behavior.
The development of advanced materials like self-healing concrete provides another dimension of contrast to the past. Historically, wooden bridges demanded a high level of routine maintenance and repair. The 21st century seeks more durable solutions to manage infrastructure, raising considerations about the philosophy behind repair and renewal as it relates to aesthetics and engineering design. We also see a growing exploration of how to replicate historical design features like curved trusses using modern CAD and computational tools. The ability to create complex geometries and stress distributions with modern software challenges us to think about the balance between historical styling and contemporary design optimization. The relationship between aesthetics and function remains a point of discussion in bridge engineering, with engineers constantly reflecting on the interplay of traditional approaches and innovative ideas that enhance design longevity and usefulness.
Structural Analysis of Woodstock's Middle Covered Bridge A Modern Take on Historic Design - Load-Bearing Capacity Analysis of Timber Trusses
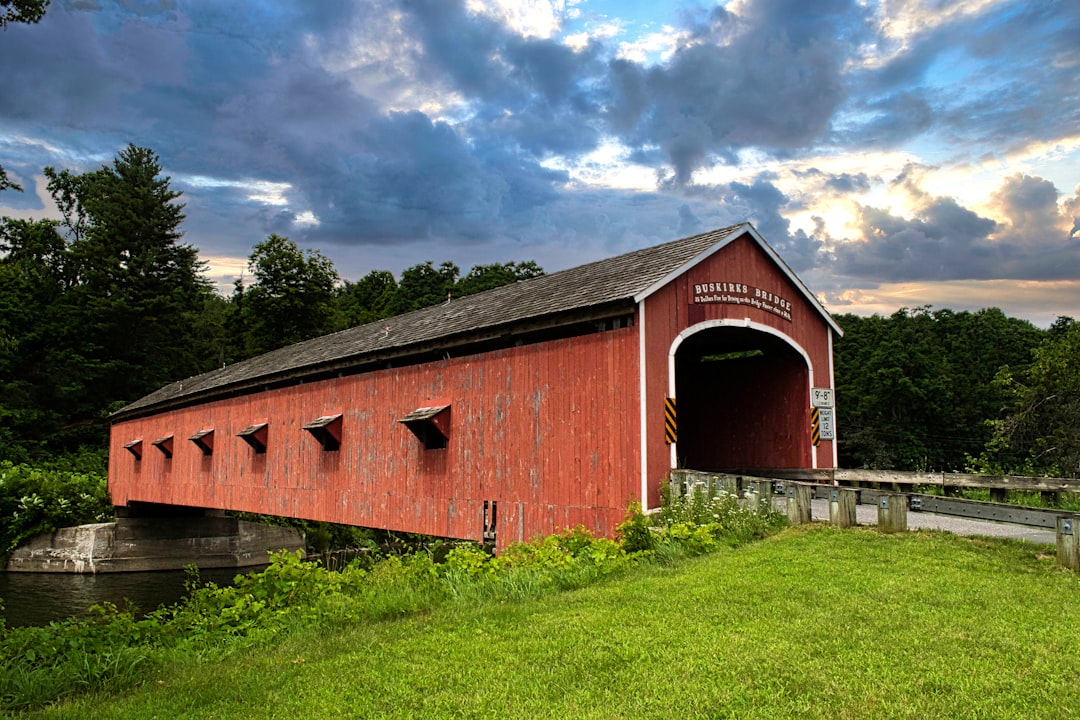
Understanding the load-bearing capacity of timber trusses is fundamental in structural analysis, especially when dealing with historic structures like Woodstock's Middle Covered Bridge. Timber, a building material with a history spanning millennia, offers cost-effective solutions for roof spans, particularly those with pitched designs, up to around 12 meters. However, timber's natural tendency to degrade over time can compromise its strength, leading to potential failure. Thus, a rigorous analysis, both through numerical modeling and physical experimentation, is crucial for assessing the structural integrity of timber components. This is especially true in ensuring that these traditional building systems meet contemporary safety regulations.
Modern approaches to structural engineering must carefully evaluate the stresses placed on timber elements, including those resulting from buckling and bending. Finding the sweet spot between preserving the artistry of historical craftsmanship and adhering to modern safety standards becomes a challenge for engineers. The need to consider these various factors – traditional construction and the complex demands of modern usage – compels us to scrutinize the dynamic forces that impact the performance of a structure. This rigorous examination helps us better appreciate the intricate interplay between legacy and innovation in a historically significant setting like Woodstock's Middle Covered Bridge.
Timber trusses, a construction method dating back millennia, have long been utilized to span wide spaces. Their load-bearing capacity, however, is highly dependent on factors like truss configuration. For example, Warren trusses, with their triangular layouts, often exhibit better strength-to-weight ratios compared to Pratt trusses due to their efficient load dispersal.
The wood species chosen also significantly impacts the overall strength. Hardwoods like oak and maple boast higher compressive strengths than softer options like pine, making material selection a key aspect of achieving optimal performance. Even the moisture content of the wood can be a major factor, with wood's natural expansion and contraction under varying humidity levels leading to potential strength reductions of up to 30%. Understanding and mitigating the effects of climate conditions is vital in the design process.
Historically, timber truss design relied heavily on empirical observations. 19th-century engineers often assessed load capacity by referencing the past performance of similar structures. While innovative, this approach lacked the detail and precision that modern methods can offer.
Thankfully, contemporary engineers leverage sophisticated computational modeling techniques to simulate loading conditions with impressive accuracy. These tools can pinpoint subtle stress points and potential failure locations within timber structures, enabling engineers to enhance safety margins far beyond the limitations of earlier methods.
Beyond their primary structural role, timber trusses offer some interesting functional advantages. Wood has a natural ability to absorb and dissipate sound, making it a beneficial material for bridges in urban areas where noise reduction is a concern. This attribute adds a further layer of consideration to the broader performance of the structure.
However, it's important to understand that timber trusses do not always experience consistent loads. The phenomenon of "shear lag" highlights that the loading distribution may not be uniform across the entire structure. This aspect necessitates detailed analysis, as a simplistic assumption of even load distribution can lead to unexpected failures if not accounted for properly.
The connections in the truss system also play a crucial role. Connections, whether traditional wooden dowels or more modern metal fasteners, directly influence the overall load-bearing capacity. Thorough consideration of joint geometry and material selection is essential to achieve robust structural integrity. Unfortunately, such intricate details can also introduce areas of weakness if not carefully designed and executed.
The inherent geometry of timber trusses—characterized by a pattern of repeating triangles—effectively distributes loads and, importantly, minimizes material usage. This efficiency makes the design inherently resourceful and can inspire innovative hybrid approaches that incorporate both wood and modern materials.
Finally, the enduring performance of some historical timber bridges, with structures lasting far longer than their projected lifespans, raises questions about our modern assumptions about structural durability. It makes one wonder if contemporary designs could benefit from insights into the surprising resilience of traditional wood structures. Is it possible that our current use of materials, particularly steel and concrete, is overlooking valuable lessons in longevity from the past? This area of research could lead to some intriguing discoveries in both the design and material selection choices we make as we move forward.
Structural Analysis of Woodstock's Middle Covered Bridge A Modern Take on Historic Design - Modern Materials Enhancing Traditional Construction Techniques
Modern materials are significantly altering the way traditional construction techniques are applied, offering advantages that blend historical construction methods with the demands of modern engineering. The incorporation of advanced materials, including glulam, high-strength steel, and concrete designed to self-repair, not only enhances durability and load capacity but also unlocks the possibility of intricate designs that echo traditional aesthetics. This merging of older and newer methods encourages a more environmentally responsible approach by promoting the use of locally sourced and sustainable materials, honoring the history of a place while also meeting present-day performance standards. It is, however, imperative to carefully consider the potential consequences of this shift, especially regarding the preservation of the valuable expertise and methods refined through generations of traditional builders. The key to the future of construction in areas with significant historical structures, like the Woodstock Middle Covered Bridge, lies in the creation of solutions that strike a balance between respecting established traditions and embracing innovation.
The field of materials science has progressed significantly, offering new avenues for enhancing traditional construction techniques, particularly in historic preservation projects like Woodstock's Middle Covered Bridge. Materials that adapt to environmental fluctuations, like temperature changes, are becoming more prevalent. These so-called "smart materials" could potentially bolster a bridge's structural integrity, contributing to a longer lifespan.
Advanced composite materials, like those using fibers reinforced with polymers, are another promising development. They boast a better strength-to-weight ratio compared to traditional materials and can distribute loads more uniformly, lessening the chances of the localized failures often seen in wooden truss systems.
3D printing technologies have also emerged as a tool to manufacture intricate components, making it possible to create complex truss connections or intricate lattice structures that would be challenging to achieve through traditional methods. This could lead to both aesthetic improvements and greater structural efficiency.
Durability is another area where advancements are being made. Nano-coatings, for instance, can provide protective layers against moisture and chemicals, thus reducing the need for constant maintenance and extending the time between repair cycles for wooden or metal parts in a bridge.
The integration of IoT-enabled sensors in modern structures allows for constant monitoring of stress, vibration, and displacement. This provides valuable real-time data for assessing structural health, enabling a proactive approach to maintenance and potentially increasing a bridge's overall service life.
Geopolymer concrete, an alternative to traditional Portland cement, presents a durable and environmentally-conscious option. Made from industrial waste products, it offers excellent resistance to many of the environmental factors that degrade wooden structures over time.
Managing vibration and dynamic loads, like those from heavy traffic or strong winds, is another area where innovation is playing a role. Technologies like tuned mass dampers are being employed to reduce oscillations, preventing structural fatigue and prolonging the lifespan of older designs that may not have been initially conceived with such forces in mind.
The combination of traditional aesthetics with modern structural capabilities has also led to "hybrid" construction. This approach leverages the strengths of steel alongside the visual appeal of wood, resulting in structures that are both resilient and historically representative.
Finite element analysis (FEA) has become more refined, offering more detailed simulations of loading conditions and the way materials interact within a structure. This allows engineers to assess the load-bearing capacity of traditional wood structures combined with modern materials with a higher degree of precision.
Finally, improvements in fastener technology have led to corrosion-resistant options with specialized coatings. This is significant because fastener failures are a primary cause of problems in both new and restored timber structures.
The ongoing development of new materials and analysis techniques offers a unique opportunity to bridge the gap between traditional building methods and the need for enhanced performance in modern settings. This is evident in the ongoing efforts to revitalize and preserve historic structures like Woodstock's Middle Covered Bridge. It will be fascinating to see how the blending of these innovations continue to reshape how we approach construction in the future.
Structural Analysis of Woodstock's Middle Covered Bridge A Modern Take on Historic Design - Environmental Impact and Sustainability of the Bridge Structure
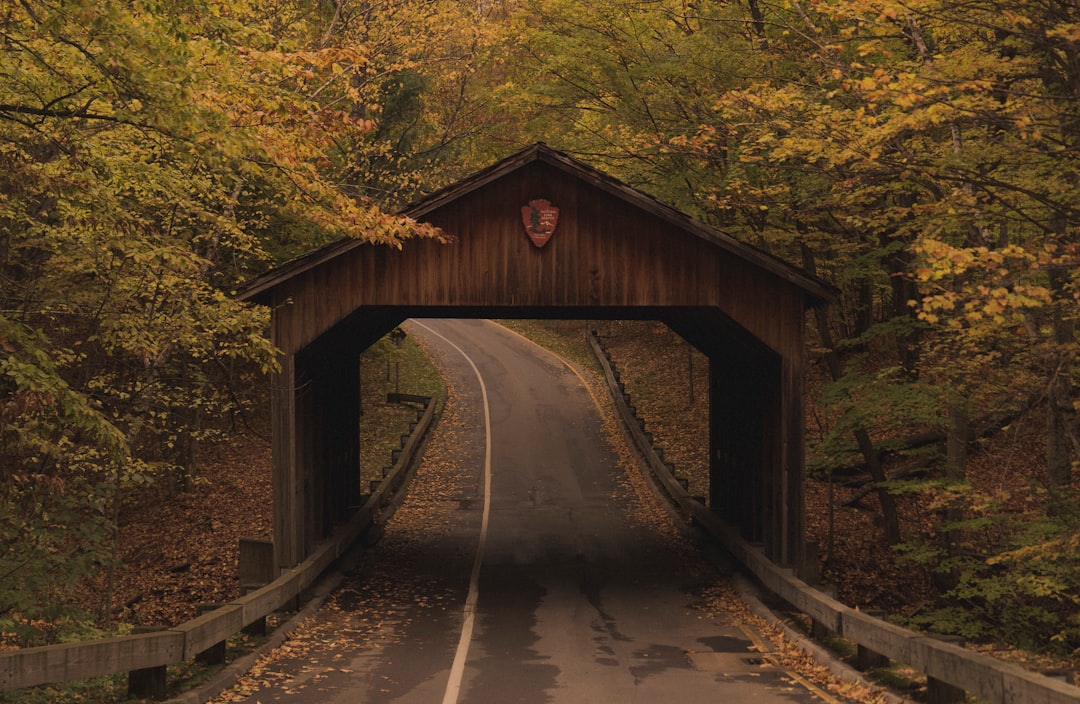
The environmental implications and long-term sustainability of bridge structures, exemplified by Woodstock's Middle Covered Bridge, are increasingly central to contemporary bridge engineering. Understanding the full environmental impact of a bridge design, from material sourcing to construction and eventual dismantling, requires a comprehensive life cycle assessment. This approach highlights the environmental benefits of certain material choices, such as timber, which studies show have a lesser impact on the environment compared to commonly used concrete across multiple environmental factors.
However, the move toward sustainability isn't solely about material choices. It requires a broader consideration of environmental and social factors, such as the impact on local communities and the long-term equity and social justice aspects of the project. This signifies that sustainability must go beyond pure structural analysis and technical considerations.
Beyond initial construction, the ability to continuously monitor and analyze structural integrity is essential. Through the use of sensors and advanced techniques, we can better understand and predict the behavior and long-term health of a structure, potentially extending its lifespan and minimizing the need for replacements or extensive repairs. This ongoing monitoring provides the ability to manage a bridge's sustainability, both in terms of minimizing its environmental footprint and preserving its historical and cultural value.
Ultimately, the drive for greater sustainability in bridge design necessitates a careful balancing act between innovative techniques and a respect for historical methods and existing infrastructure. Finding this balance ensures that future generations can benefit from the same enduring value and structural integrity we find in Woodstock's Middle Covered Bridge, while minimizing any negative environmental consequences.
The Woodstock Middle Covered Bridge exemplifies how timber's inherent properties influence structural design. While timber excels in compression, its relatively weaker tensile strength necessitates careful planning of truss configurations to maximize load-bearing capacity. Intriguingly, wood's natural sound-dampening ability could enhance the user experience, making timber bridges quieter than those built with steel or concrete, especially in high-traffic zones. This becomes a key factor for urban environments where noise pollution is a concern.
Unlike the potential for catastrophic failure in steel under certain conditions, wood typically degrades gradually, providing visible signs of weakening that can guide maintenance schedules. This characteristic offers valuable early-warning indicators of potential issues, suggesting a proactive maintenance approach. Contrary to assumptions, certain hardwoods, like hickory, exhibit superior load-bearing capacity and durability when compared to softer options like pine. Material selection thus becomes a critical factor when preserving historical bridges, ensuring the optimal choice for strength and long-term performance.
Wood's susceptibility to moisture fluctuations, leading to strength reductions of up to 30%, underscores the importance of environmental considerations in the design process. Even subtle shifts in humidity can drastically affect a bridge's performance, demanding close attention from engineers. Timber truss designs exhibit remarkable efficiency through the use of triangles. This geometric arrangement not only optimizes load distribution but also minimizes material usage, a prime example of resourceful design practices.
Research suggests that traditional woodworking techniques, such as mortise and tenon joints, may outperform modern mechanical fasteners in both strength and flexibility. This challenges the notion that modern approaches are automatically superior and highlights the value of traditional knowledge. Many historical timber bridges have surpassed their projected lifespans, leading us to question our assumptions about material and design longevity compared to time-tested wooden structures. Could our reliance on modern materials like steel and concrete be neglecting valuable lessons from the past? This is a fruitful area for further investigation.
Timber possesses viscoelastic characteristics, effectively absorbing and dissipating energy during dynamic events like strong winds or vehicle impacts. This makes it a desirable material in areas prone to seismic activity when compared to more rigid materials. Shear lag, an aspect of load distribution within a truss system, reveals the complex nature of timber structures. Uneven load distribution can lead to unexpected failures if not carefully accounted for during the design phase, emphasizing the importance of detailed analysis. Understanding the phenomenon of shear lag is crucial to ensuring the long-term stability of these structures.
Structural Analysis of Woodstock's Middle Covered Bridge A Modern Take on Historic Design - Preservation Challenges and Solutions for Historic Bridge Features
Maintaining the unique features of historic bridges presents a complex balancing act. We need to respect their historical value while also meeting modern engineering and safety standards. This challenge is particularly acute when dealing with bridges like Woodstock's Middle Covered Bridge, where preserving the original design must be weighed against the need for enhanced structural integrity to handle current traffic loads and safety standards. Many bridges nationwide are in danger of being classified as structurally deficient, emphasizing the urgent need for clear guidelines on how to maintain and restore historic bridges. This is where collaboration plays a crucial role, with input from State Historic Preservation Offices and other stakeholders being essential to make informed decisions that respect both engineering principles and the rich historical context these bridges embody. The path forward requires finding solutions that incorporate modern materials and techniques where necessary, while upholding the essence of the original craftsmanship. By integrating modern knowledge with the historical methods, we can better ensure these structures continue to serve as valuable landmarks for future generations.
A considerable portion of our nation's bridges are flagged as structurally deficient or obsolete, a concerning trend that underscores the need for improved safety standards. There's a clear call for a comprehensive national framework that guides the preservation and restoration of historical bridges, balancing engineering principles with environmental concerns. While the existing Historic Bridge Preservation Guide outlines procedures for both fixed and movable bridges, it doesn't comprehensively address mechanical and electrical aspects. Woodstock's Middle Covered Bridge, Vermont's first state-constructed covered bridge since the late 19th century, replaced an older iron bridge built in 1877. Interestingly, this 1969 bridge was built by Milton Graton and had a unique transportation journey across the river using a team of oxen.
The National Historic Covered Bridge Preservation Program, established in 1998, is a focused effort to develop reliable techniques for restoring and replacing these valuable historical assets. One of the key challenges for historic bridge preservation is finding that delicate balance between respecting their historical significance and satisfying modern engineering and safety requirements when making decisions about their future. State Historic Preservation Offices (SHPOs) and other stakeholders are integral partners in shaping the decisions regarding preservation, restoration, rehabilitation, or replacement of these bridges.
In a real-world example, the Carrollton Bridge in Indiana provides valuable insights through its case study. Its historical significance as the first enduring crossing of the Wabash River showcases the significance of context when evaluating bridge preservation strategies. The knowledge accumulated from transportation departments involved in these types of projects holds the potential to shape the way we approach the future of historic bridges. Experiences gained through these bridge projects provide critical lessons and best practices that inform our approach. It's clear that the complex interactions between past methods and contemporary demands will play a significant role in the long-term preservation and management of our remaining historical bridge infrastructure.
Structural Analysis of Woodstock's Middle Covered Bridge A Modern Take on Historic Design - Digital Modeling and Simulation in Structural Assessment
Digital modeling and simulation are increasingly crucial for understanding the structural behavior of historic bridges like Woodstock's Middle Covered Bridge. These tools allow engineers to create virtual representations of the bridge, capturing intricate details and enabling in-depth analysis. By generating digital twins from various sources, including point cloud data, engineers can pinpoint areas of weakness within the existing structure and determine where reinforcement is needed. Techniques like Building Information Modeling (BIM) and Finite Element Analysis (FEA) have become integrated into the assessment process, offering precise evaluation of stresses and loads, helping to understand how the bridge responds to various forces. This information is vital when deciding on preservation strategies that balance historic design with modern safety standards. Furthermore, the integration of advanced monitoring technologies and automation in structural health monitoring offers continuous oversight, enabling a proactive approach to maintaining the long-term stability and safety of these historic structures. While the use of these modern technologies offers a significant advantage in bridge assessment, the delicate dance of integrating innovation into historical structures demands careful attention to preserving traditional craftsmanship and techniques. This careful balance will be crucial for ensuring the continued structural integrity and preservation of these historically important bridges.
The application of digital modeling and simulation has revolutionized the way we assess the structural integrity of historic structures, particularly bridges like Woodstock's Middle Covered Bridge. Digital twin technology allows for real-time monitoring and predictive analysis, providing a more proactive approach to maintenance compared to traditional methods. For example, engineers can now visualize potential stress points and areas prone to failure, enabling interventions before issues escalate.
Finite element analysis (FEA) offers a level of precision that was previously unimaginable. Through simulations, we can now understand how the intricate components of a bridge interact under varying loading conditions, revealing subtle material behaviors that were hidden using traditional approaches. This increased understanding enhances not only structural safety but also leads to more efficient designs.
Parametric design tools offer a fascinating opportunity to explore a wider range of design possibilities. By altering variables within a model and instantly seeing the impact, engineers can find innovative solutions that bridge the gap between historical aesthetics and modern performance requirements. This capability is particularly helpful in balancing the need to preserve a structure's character with the demands of modern engineering.
The integration of sensors and the Internet of Things (IoT) has also significantly improved our ability to monitor structures. Structural health monitoring (SHM) systems provide continuous data on vibration, displacement, and stress levels, allowing for early detection of potential issues. This level of continuous monitoring is a major advantage over traditional methods, which often rely on visual inspections that can miss subtle indicators of deterioration.
Advanced computational models enable us to analyze how dynamic loads from traffic and environmental conditions influence bridge behavior. This is especially useful for evaluating historic structures like covered bridges, where understanding live loads is critical for ensuring structural integrity over time. These models also offer deeper insights into the behavior of materials, like timber, revealing how it performs under complex load combinations and environmental conditions.
Moreover, the ability to simulate nonlinear material responses provides a more nuanced understanding of how timber interacts with forces and environmental factors. This is critical for predicting potential failure modes in older structures where timber might have experienced degradation or weakening over time.
Augmented reality (AR) presents yet another opportunity to visualize complex data and models in a more intuitive way. Engineers can overlay AR elements onto a physical bridge, providing a comprehensive understanding of design changes and potential structural weaknesses in a way that promotes collaboration and communication.
Digital modeling has become essential for meticulously documenting and simulating historic preservation techniques. This ensures that any restoration or modification respects the integrity of the original design while incorporating modern standards for safety and durability.
Furthermore, digital tools are revealing hidden aspects of traditional joinery and construction techniques that can be replicated with modern technologies. This raises thought-provoking questions about the potential for traditional craftsmanship to co-exist with the advancements of digital fabrication.
Finally, the capacity to conduct virtual resilience testing is a valuable tool for assessing how bridge designs might react to extreme weather events, like floods or earthquakes. This predictive capability is particularly important when considering historic structures that may not have been designed with today's environmental challenges in mind.
In conclusion, digital modeling and simulation are instrumental in preserving the valuable legacy of historic structures like the Woodstock Middle Covered Bridge while simultaneously ensuring their structural safety and integrity in modern times. It will be exciting to see how these technologies continue to refine our understanding of historic structures and inform future restoration and preservation efforts.
More Posts from aistructuralreview.com: