Washington's Evolving Seismic Design Standards A 2024 Update for Structural Engineers
Washington's Evolving Seismic Design Standards A 2024 Update for Structural Engineers - Updated Snow and Rain Load Provisions in 2024 IBC
The 2024 International Building Code (IBC) brings about revisions to how snow and rain loads are addressed in structural design. These changes, largely driven by aligning with the ASCE 7-22 standards, introduce a new approach to calculating rain loads. This approach considers factors like static, hydraulic, and ponding heads, aiming for a more comprehensive understanding of potential water loads. Moreover, the IBC now incorporates risk categories for various loads, moving away from uniform requirements and adjusting for the specific hazards faced by individual structures. It's important to note that the 2024 edition, for the first time, specifically acknowledges the need to design for tornado loads, highlighting the increasing concern about severe weather events. These updated provisions aim for enhanced consistency and accuracy in assessing structural loads, emphasizing the need for structural engineers to thoroughly familiarize themselves with these adjustments to ensure continued design integrity and public safety across varied geographical regions and building types. It remains to be seen how effectively this new framework will be implemented, but it represents a step towards addressing evolving weather patterns and building risks.
The 2024 International Building Code (IBC) has revised its snow and rain load provisions, aligning them more closely with the ASCE 7-22 standards. One notable aspect is the introduction of new snow load maps that better capture the variations in snowfall across different locations, especially at high altitudes where past estimates may have been too low. This update appears to address the observed trend of increased snowfall, particularly in the Cascades, with corresponding increases in minimum snow load values.
Furthermore, the focus on rain load calculations has intensified. This shift recognizes the increasing frequency and severity of extreme rainfall events, demanding more sophisticated analyses that incorporate the impact of static, hydraulic, and ponding heads to calculate water loads. We're seeing a greater emphasis on combined snow and rain loads, prompting a need for more comprehensive testing of structural components. The IBC now requires engineers to address potential 'rain-on-snow' scenarios, which can significantly increase roof loads quickly.
These changes also address the crucial but often overlooked role of roof drainage systems, suggesting a move towards designs capable of handling the greater runoff volumes expected with more intense rainfall and melting snow. The inclusion of ice formation as a load factor is somewhat unexpected, highlighting the need to evaluate how ice accumulation on structures can contribute to loading, potentially impacting standard calculations.
The updated code also advocates for more advanced tools like GIS to create region-specific snow load maps, readily available for design use. This increased reliance on technology extends to real-time weather data, suggesting a growing acceptance of adaptive engineering practices that can accommodate immediate weather conditions.
It's also intriguing to note that the 2024 IBC, in certain cases, allows for performance-based design in calculating snow and rain loads. While offering greater flexibility, this approach does require a deeper level of engineering expertise to execute correctly. While the intent is certainly to promote consistency in design across locations and structure types, the application of these updates will undoubtedly lead to more nuanced and complex design considerations for structural engineers. The IBC has made these new provisions accessible through online resources, presumably intending to facilitate their adoption by engineers and building officials alike.
Washington's Evolving Seismic Design Standards A 2024 Update for Structural Engineers - Risk-Based Approach for Environmental Loads
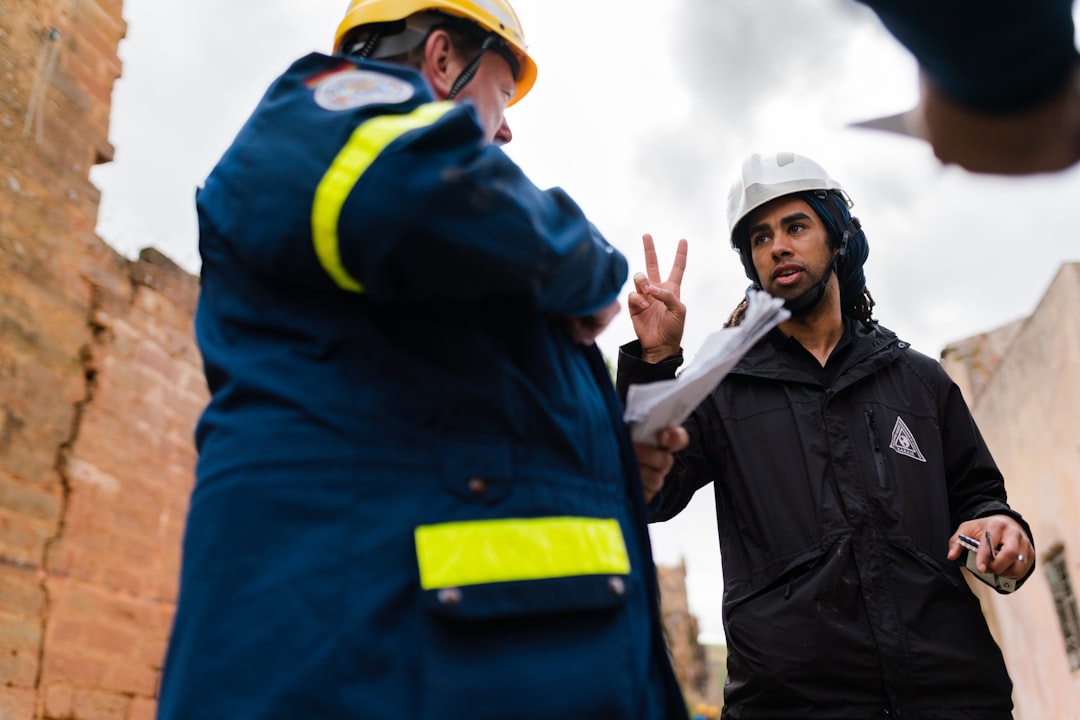
Washington's evolving seismic design standards, as reflected in the 2024 updates, now emphasize a risk-based approach to environmental loads. This shift recognizes that building safety isn't just about withstanding earthquakes, but also about managing the increasingly complex interplay of environmental forces. This approach acknowledges that structures can be affected by a combination of factors, including seismic activity, severe weather, and climate change.
The new risk-based perspective necessitates a more sophisticated understanding of how these various loads interact. Designers are no longer simply applying uniform standards, but rather tailoring their approach based on the specific risks posed to individual structures. This often involves incorporating risk categories that classify structures based on their exposure to potential hazards. Furthermore, a move towards performance-based design allows engineers to develop more customized solutions to ensure adequate resilience.
This updated approach challenges some of the long-held assumptions in traditional load-resistance factor designs. It encourages a more dynamic and context-sensitive perspective, where risk is assessed in relation to the specific building and its environment. Given the growing frequency and intensity of severe weather events, it's critical that building design practices adapt to these emerging realities. The future of structural safety in Washington hinges on successfully incorporating this new risk-based paradigm.
The shift towards a risk-based approach for environmental loads suggests a move away from solely relying on prescriptive design methods towards a more performance-driven approach. This allows engineers to tailor designs based on site-specific risk assessments instead of adhering to uniform code requirements. This approach incorporates probabilistic modeling to better understand the likelihood of various environmental loads, such as earthquakes and flooding, thereby facilitating a more effective approach to anticipating and minimizing structural risks.
It's intriguing that the updated IBC acknowledges the need to design certain structures, such as hospitals and emergency response facilities, to higher performance standards because of their crucial role during disaster situations. This highlights a departure from traditional load considerations, where all buildings were treated equally regardless of their importance.
Within the context of seismic design, the risk-based approach emphasizes a deeper understanding of how geotechnical factors like soil type and stability influence a building's response to seismic events. This often necessitates more detailed studies into soil-structure interaction, underscoring the complex interplay between the ground and the built environment.
One notable trend is the integration of sophisticated data analytics and machine learning into risk assessments. This provides structural engineers with novel tools to evaluate historical data and predict future loading scenarios, offering a new level of precision in understanding environmental forces.
It's perhaps surprising that the new risk categories necessitate not only revisions to load factors but also demand regular updates to load calculations as new data emerges. This emphasizes the dynamic nature of environmental risks, which may fluctuate over time due to climate change or other unforeseen factors.
Furthermore, the 2024 updates anticipate a broader range of variability in environmental loads driven by changing climate patterns. Designs now need to consider adapting to more extreme conditions over a structure's lifespan, moving away from the relatively static load assumptions traditionally employed.
One of the less expected aspects of the updated code is the comprehensive consideration given to secondary load effects, such as the influence of water accumulation on structural integrity during extreme weather events. These effects can significantly impact the overall safety and stability of a structure, revealing a growing concern about the consequences of combined loading conditions.
The emphasis on implementing real-time monitoring systems in structural design could fundamentally change how engineers manage risk and conduct maintenance. This approach encourages a continuous evaluation of structures under variable environmental conditions, enabling a more proactive response to potential threats.
Interestingly, the updated code promotes a more collaborative approach by integrating stakeholder input into the risk assessment process. This encourages greater collaboration among engineers, architects, and community planners to tackle unique localized risks associated with environmental loads, fostering a more holistic and locally-responsive approach to design.
Finally, while the intentions behind these evolving standards are largely positive, there is a risk that these more complex and variable design requirements could introduce new challenges to the implementation process. It remains to be seen how the engineering profession will adapt to these intricate revisions and whether these changes result in a demonstrable improvement in building safety and resilience.
Washington's Evolving Seismic Design Standards A 2024 Update for Structural Engineers - Revised Seismic Design Category Maps
The 2024 updates to the Seismic Design Category (SDC) maps for Washington are based on the 2020 NEHRP Recommended Seismic Provisions, a significant shift for new construction and structural design. These updated maps, now officially part of both the International Residential Code (IRC) and the International Building Code (IBC), reflect a revised understanding of seismic hazards across various locations within the state. A key change is the inclusion of default site classifications, which provide a standardized method for engineers to assign the appropriate SDC for buildings based on their location's specific geological features. Furthermore, the adoption of a multi-period response spectra specifically for non-structural components suggests a growing awareness of the importance of securing non-structural elements in earthquake zones. While these revised maps bring enhanced accuracy in seismic hazard assessment, structural engineers are faced with adapting to new procedures that may alter design practices. This adaptation will likely lead to designs that ultimately enhance building resilience to seismic threats.
The revised Seismic Design Category (SDC) maps, developed using the 2020 NEHRP Recommended Seismic Provisions, represent a significant shift in how we understand and address earthquake risk in building design. They're not just about defining hazard zones, but incorporate a wider array of data, including geological, seismic, and geographical information, to pinpoint regions that were previously underestimated. This could lead to more in-depth scrutiny of structures located in these newly classified areas, requiring engineers to re-evaluate their safety margins.
The shift towards using more advanced modeling techniques is essential, as the updated maps incorporate a wider range of data types. This includes historical earthquake occurrences, soil characteristics, and projected ground motion. Consequently, engineers need to develop expertise in contemporary software tools to fully understand and interpret this more complex dataset when designing buildings.
Interestingly, these revised maps specifically identify "liquefaction-prone" zones, a factor that often was overlooked in older design standards. Regions susceptible to soil liquefaction due to strong shaking are now explicitly indicated, which underscores the critical need for tailored foundation designs in these particular locations.
One noteworthy aspect of this update is the increased frequency of map revisions, a reflection of ongoing research and continuous advancements in seismic science and technology. As a result of this evolution, structures are subject to more rigorous standards, prompting a greater emphasis on ensuring that building practices are constantly aligned with the latest findings in geological studies.
The complexity of seismic force analysis has increased substantially. The updated maps base construction criteria on probabilistic seismic design, which involves estimating the likelihood of future seismic events, rather than solely relying on historical records. This approach empowers engineers to make more informed design decisions, balancing potential risks and uncertainties.
A key element of the revised maps is their incorporation of multiple seismic source models, allowing for a more comprehensive range of earthquake scenarios. This more nuanced approach acknowledges that seismic risk can stem from a variety of sources, leading to more robust design considerations for buildings with diverse purposes.
There's also a growing awareness within the field that earthquake activity and infrastructure resilience are interconnected. This growing understanding is pushing engineers to embrace design practices that factor in cumulative risks, moving away from solely considering single-event occurrences. It's indicative of a paradigm shift in how seismic hazards are managed.
A valuable feature of these updated maps is their integration with Geographical Information Systems (GIS) technology, supplying engineers with powerful visualization tools. These maps help provide a clearer understanding of how local geological conditions and soil types interplay with seismic threats, leading to enhanced site-specific design strategies.
The revised seismic maps emphasize areas with a unique history of earthquakes, considering not only the severity of past events, but also their frequency. This historical perspective provides critical context for planning and design, prompting engineers to broaden their thinking beyond just raw risk calculation.
Ultimately, these updated seismic design categories have broad implications, fostering increased collaboration between engineers, geologists, and urban planners. This integrated approach has the goal of achieving a more resilient built environment through shared understanding and collaboration in seismic risk management. It's crucial for the future of construction.
Washington's Evolving Seismic Design Standards A 2024 Update for Structural Engineers - New Multiperiod Response Spectra for Structural Design
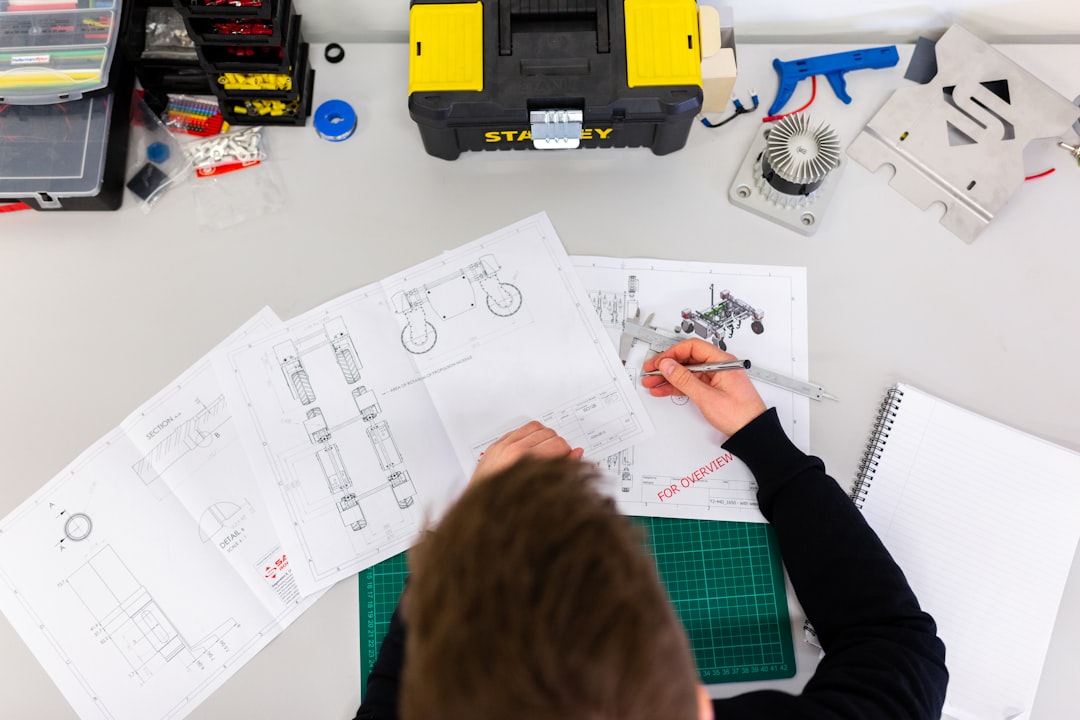
The 2024 updates to Washington's seismic design standards introduce a new approach to structural design: the Multiperiod Response Spectra (MPRS). This represents a significant shift, as MPRS consider a wider range of ground motion behaviors during a major earthquake, specifically across 22 different response periods. This contrasts with previous methods that often focused on a limited set of earthquake scenarios. The MPRS framework is now a core part of the latest seismic design provisions and related ASCE standards.
The adoption of MPRS emphasizes a deeper understanding of how structures respond to earthquakes across different frequencies of vibration. This approach requires a more detailed analysis of site-specific conditions to ensure that design considerations appropriately address the potential impacts of earthquakes on various structures.
However, this move to MPRS also presents a challenge. It demands a more sophisticated level of engineering expertise. Structural engineers will need to learn how to apply these new spectra in their designs, which might require new software and techniques. The traditional approaches may not always be sufficient. Ultimately, these updates are aimed at making structures in Washington more resilient to earthquakes. Whether it truly succeeds will depend on the effective implementation and application of these updated standards by the engineering community. The future of seismic safety in Washington now involves navigating this more detailed, complex approach.
The 2024 updates to Washington's seismic design standards introduce a new multi-period response spectrum (MPRS), which acknowledges the changing nature of earthquake forces over time. This updated approach isn't just about a structure's primary vibration frequency, but incorporates higher modes of movement too. This more detailed view of a building's dynamic response potentially leads to more accurate assessments of how it will behave during an earthquake, including how different parts of the structure interact.
This revised way of looking at seismic forces allows for a better understanding of how soil conditions affect a structure's performance. Historically, soil-structure interaction has been a significant contributor to the way buildings react in earthquakes, especially in areas with diverse soil types. The MPRS framework seems to be geared towards addressing this better.
It's noteworthy that these guidelines now require engineers to think about not only structural components but also non-structural elements when defining performance criteria. This recognizes the crucial role these secondary elements play in overall building safety during a quake.
The enhanced modeling behind the new response spectra signals a big shift from the traditional reliance on observations. Now, more sophisticated simulations are being used to account for a range of potential future scenarios, making the design process more robust and acknowledging uncertainties in earthquake behavior.
The integration of MPRS into the design process seems to be in line with the broader trend towards performance-based engineering. This focus on desired outcomes during earthquakes, rather than just compliance with a set of standards, should improve safety.
Adapting to this new approach is a challenge to traditional design practices. It requires engineers to step away from relying on standard solutions and to start thinking more about site-specific conditions. This will likely lead to designs that are more nuanced and locally tailored to each structure's unique circumstances.
Because local geological conditions have a large effect on seismic performance, the prominence of MPRS suggests that engineers will need to work more closely with geologists when evaluating sites and designing.
Interestingly, the increased complexity of load calculations and design criteria might also lead to some confusion among engineers. It's possible that further training and educational materials might be needed to ensure a smooth transition to this new system.
While these updated standards hold the promise of enhanced building resilience and safety, they also place greater demands on engineers. The shift to this more complex system will necessitate investment in more advanced software tools and training to properly utilize these updated methods. It will be interesting to see how the engineering community absorbs and implements this new approach and if it does in fact lead to demonstrably safer structures.
Washington's Evolving Seismic Design Standards A 2024 Update for Structural Engineers - Changes in Nonstructural Anchorage Requirements
Washington's updated seismic design standards for 2024 bring a renewed focus on how nonstructural elements, like partitions, ceilings, and equipment, are secured within buildings. This shift emphasizes that these components, often overlooked in the past, play a crucial role in a structure's overall safety and functionality during an earthquake. The new standards, aligned with ASCE 7, demand a more thorough assessment of how these nonstructural elements respond to earthquake forces.
The most notable change is the increased emphasis on dynamic analysis for certain nonstructural elements. Previously, a simpler approach was often sufficient. Now, engineers might need to analyze how these elements behave under dynamic loading conditions, considering factors like the component's material properties and its connection to the primary structure. Additionally, updated provisions address the significant impact of the inherent flexibility or rigidity of these nonstructural elements, which affects how they respond to seismic shaking. This new consideration acknowledges that some components might be more prone to damage depending on their design and material.
Ultimately, these changes aim to improve the overall resilience of buildings by demanding a more robust and comprehensive approach to nonstructural anchorage. This increased attention to detail in the design of nonstructural components is intended to minimize damage, protect occupants, and ensure that buildings can continue to function after a seismic event. However, it also places a higher burden on engineers, requiring them to adopt more sophisticated methods and analysis techniques for their designs.
Seismic design for nonstructural components has seen significant changes, especially with the shift away from older standards like the Uniform Building Code (UBC) towards newer ones like ASCE 7 and ACI 318. The 2015 International Building Code (IBC) now mandates that these elements must withstand earthquake forces according to ASCE 7 guidelines. This includes a focus on equations and factors within ASCE 7 Chapter 10 for calculating those forces.
Concrete structures, specifically, rely on ACI 318-11 (and its later update) for anchor design in seismic situations, particularly in high-risk seismic areas (C, D, E, or F). The design of anchors for nonstructural elements within concrete follows specific rules aimed at resisting earthquake forces. Notably, the ASCE 7 Supplement 1 provides specific values for something called a response modification factor (Rp) that can differ considerably based on the characteristics of the nonstructural element being designed.
A key aspect of how nonstructural elements react during seismic events is their flexibility or rigidity. This is determined by their fundamental period (Tp), and the Tp greatly impacts the forces they experience in an earthquake. We're also seeing a big change in how nonstructural anchorages are designed. Dynamic analysis is being pushed more now, and this leads to adjustments in what are called ap factor values, which are part of the force calculations. These values change based on how we expect the nonstructural element will behave.
The upcoming 2024 updates will reflect these trends. They provide new guidelines for the design and anchoring of these types of components within Washington's seismic standards. There's been a strong emphasis from key players in this field, and the various committees involved in these standard revisions, on collaboration to ensure the provisions work effectively across a broad range of locations. There's a real sense that this is a process that needs collaboration from multiple engineering fields to make sure what gets adopted in Washington's standards can be applied broadly and safely across different locations in the region.
Washington's Evolving Seismic Design Standards A 2024 Update for Structural Engineers - Horizontal Force Design for Structural Frames and Walls
The 2024 updates to Washington's seismic design standards reflect a significant shift in how horizontal forces are addressed in structural frames and walls. These revisions emphasize the critical role of connections designed to resist these forces, particularly aligning with the requirements outlined in ASCE 7. The importance of shear walls, especially in masonry structures, as crucial elements for resisting in-plane loads remains paramount. The new standards delve deeper into the details of shear wall design, refining both strength and seismic detailing requirements. This ensures that structures can better withstand the stresses imposed by seismic events, contributing to enhanced stability.
A notable trend in these updates is the increasing adoption of risk-based and performance-oriented design principles. This signifies a move away from solely relying on prescriptive methods, encouraging engineers to consider site-specific conditions and tailor their designs accordingly. This shift towards more nuanced and contextual design approaches, although beneficial, necessitates a heightened level of technical understanding from the engineering community. Effectively navigating these updated standards will be crucial to ensuring that structures in Washington are adequately prepared to face the dynamic nature of seismic hazards. While these evolving standards offer a more sophisticated and resilient approach to seismic design, the increased complexity may present challenges that engineers will need to overcome.
The 2024 updates to Washington's seismic design standards place a strong emphasis on how structural frames and walls handle horizontal forces. This shift reflects a broader awareness that building stability is influenced not only by earthquakes but also by severe weather patterns, prompting the need for a more holistic perspective on environmental loads.
These standards encourage a more comprehensive approach to load calculations, recognizing that structures can experience combined effects from both lateral seismic forces and various environmental hazards. This transition often requires engineers to develop more complex mathematical models to represent these interacting loads, going beyond simpler approaches of the past.
One intriguing observation within these updates is the acknowledgement that during seismic events, forces redistribute within frames and walls in ways that can deviate from static assumptions. This realization underscores the need for dynamic analysis, where we consider the time-varying nature of these forces, to predict structural response more accurately.
To better model real-world scenarios and account for this complex force redistribution, the latest standards encourage the use of sophisticated computational simulations. This transition inevitably requires engineers to gain familiarity with advanced software that can capture these dynamic behaviors, prompting a need for ongoing skill development and training.
There's a clear move towards performance-based design, where solutions are tailored to specific loading scenarios. This shift in approach, however, means designs could deviate significantly from the more prescriptive methods previously employed. This introduces a challenge to engineers as they must learn how to integrate these more customized solutions into their practice.
Furthermore, the new standards highlight the significance of how nonstructural elements, such as partitions and ceilings, interact with main frame systems during earthquakes. This emphasizes that the design and anchoring of these often-overlooked components are vital to a building's ability to resist horizontal forces and minimize damage during seismic events.
These updated provisions also acknowledge the complex interplay between the ground and the structure, which is particularly important in regions susceptible to liquefaction. This necessitates a more thorough understanding of soil-structure interaction, highlighting the need for detailed geotechnical studies to inform design decisions.
The definitions and applications of importance factors, especially for structures in high-risk areas, have been revised. This has implications for existing buildings, possibly necessitating renovations to meet the new horizontal force design criteria, which is an area ripe for future research and clarification.
Another important shift is the increased emphasis on collaboration. The revisions advocate for a more interdisciplinary approach, recognizing that effective solutions for horizontal force design require input from a variety of professionals, including structural engineers, geologists, and urban planners.
Finally, the rapid pace of change in seismic design standards necessitates continuous learning and adaptation for engineers. Staying current with the latest methodologies and technologies is crucial for effectively mitigating the risks posed by various horizontal load conditions. It's a continual process of education and refinement that promises to continue shaping the future of structural design in Washington and beyond.
More Posts from aistructuralreview.com: