Revolutionize structural engineering with AI-powered analysis and design. Transform blueprints into intelligent solutions in minutes. (Get started for free)
Análisis Comparativo Eficiencia y Sostenibilidad de Elementos Estructurales en Edificaciones Modernas
Análisis Comparativo Eficiencia y Sostenibilidad de Elementos Estructurales en Edificaciones Modernas - Comparing Steel and Concrete Structural Elements Efficiency
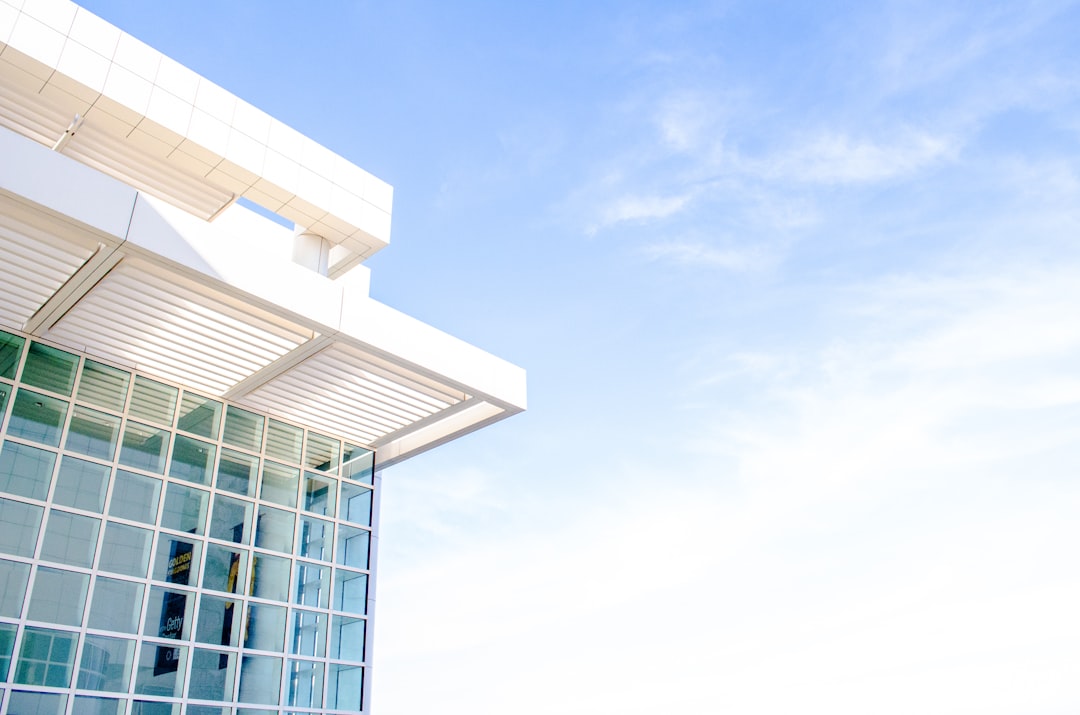
When comparing the efficiency of steel and concrete structural elements, a nuanced understanding of their advantages and disadvantages is essential. While concrete's thermal mass can provide energy savings by regulating temperature fluctuations, the environmental impact of its production, including higher emissions and energy consumption, cannot be overlooked. Steel, on the other hand, boasts superior load-bearing capacity and seismic resistance, enabling faster construction and greater design flexibility, albeit often requiring additional concrete support for stability. A comprehensive analysis considers initial costs, long-term durability, environmental impact, and specific project requirements to determine the most efficient structural solution for modern building practices. It is critical to acknowledge the interconnectedness of these factors and prioritize informed decision-making to ensure both structural integrity and sustainability in building design.
The comparison of steel and concrete in structural applications reveals a fascinating interplay of strengths and weaknesses. Steel, with its superior tensile strength, allows for slender and elegant designs, especially where stretching forces are prevalent. This translates to lighter structures, potentially reducing foundation needs and construction time. While concrete boasts inherent fire resistance, advancements in steel treatment and coatings have brought it on par in terms of fire safety.
However, the ductility of steel offers a significant advantage in seismic zones. Its ability to deform under stress before failure is crucial for withstanding lateral forces. Concrete's compressive strength makes it a strong choice for load-bearing applications, but its brittleness poses a risk of sudden failure, contrasting with the more gradual yielding of steel.
Lifespans vary considerably. Steel, with proper maintenance, can reach a century, while concrete often requires repairs after 30-50 years due to corrosion or cracking. Steel's fabrication process, with its tighter tolerances, lends itself to more precise construction, potentially improving structural performance. Its cold-weather adaptability, unlike concrete which can be affected by low temperatures, makes it a preferred choice in colder climates.
Furthermore, repairing damaged steel components is typically faster and less labor-intensive compared to the more involved process of concrete repairs. Steel's unmatched recyclability further enhances its sustainability credentials. In contrast, recycling concrete poses significant challenges, highlighting steel's position as a reusable resource.
While concrete's initial material costs are often lower, steel emerges as a more efficient option in the long run due to faster construction times and its greater durability. This comparative analysis underscores the importance of considering a project's specific needs and environmental context when making the choice between these two fundamental structural materials.
Análisis Comparativo Eficiencia y Sostenibilidad de Elementos Estructurales en Edificaciones Modernas - Energy Performance of Prefabricated vs On-Site Construction
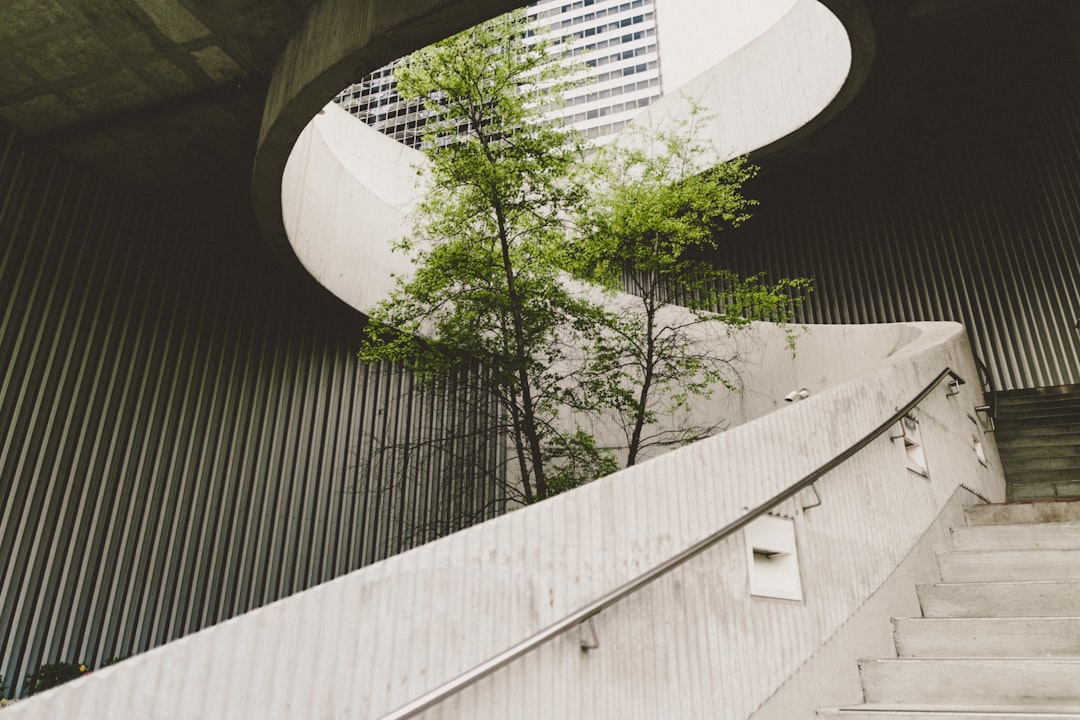
The energy performance of prefabricated construction (PFC) is a crucial aspect of the conversation about modern building practices. While traditional on-site construction has been the norm, PFC offers advantages in terms of energy efficiency and environmental impact. Prefabricated buildings are generally built with less material waste and require less energy during transportation and construction. This reduced energy demand translates to a lower carbon footprint compared to on-site construction. Furthermore, the inherent precision of prefabrication leads to greater design accuracy, contributing to a more efficient building performance.
The move towards prefabricated construction demonstrates a growing awareness of the need for more sustainable building practices. As the industry seeks solutions for reducing energy consumption and environmental impacts, PFC is increasingly recognized for its potential to address these challenges.
Prefabricated construction, with its factory-based production of building components, has emerged as a potential solution to enhance energy efficiency in the construction sector. This method can significantly improve a building's overall energy performance, potentially exceeding that of traditionally built structures.
A key advantage is the controlled environment of the factory setting, which enables the precise application of materials and the integration of energy-efficient features right from the start. This level of precision can lead to more consistent thermal envelopes and fewer gaps or thermal bridges compared to on-site construction, where variations in weather and site conditions can impact the effectiveness of insulation and other energy-saving measures.
Numerous studies have revealed the positive impact of prefabrication on energy consumption during the construction phase, with some studies even suggesting up to a 50% reduction in energy costs. This reduction stems from optimized material use, reduced waste, and streamlined logistics, resulting in lower energy consumption for material transport and on-site activities.
Prefabricated methods also offer the advantage of quicker construction timelines. This is not only beneficial for project completion but also for minimizing energy expenditure related to site management, utilities, and overall site operations during the construction period.
Moreover, the prefabrication approach allows for the incorporation of advanced insulation materials and energy systems into the building’s design during the factory production stage. This proactive integration can significantly boost the building’s energy performance from day one.
Interestingly, the initial costs associated with prefabricated buildings, while often perceived as higher, can be offset by long-term cost savings. Reduced energy consumption during operation, stemming from improved insulation, efficient building design, and integration of energy-saving technologies, can contribute to lower overall lifetime costs.
While prefabrication offers a compelling approach to energy-efficient building construction, it is crucial to acknowledge that the actual energy performance of prefabricated buildings can vary widely based on factors such as design, materials, and construction practices. A thorough analysis of these factors is essential for optimizing energy performance and maximizing the benefits of prefabrication.
Análisis Comparativo Eficiencia y Sostenibilidad de Elementos Estructurales en Edificaciones Modernas - Seismic Resilience of Advanced Structural Systems
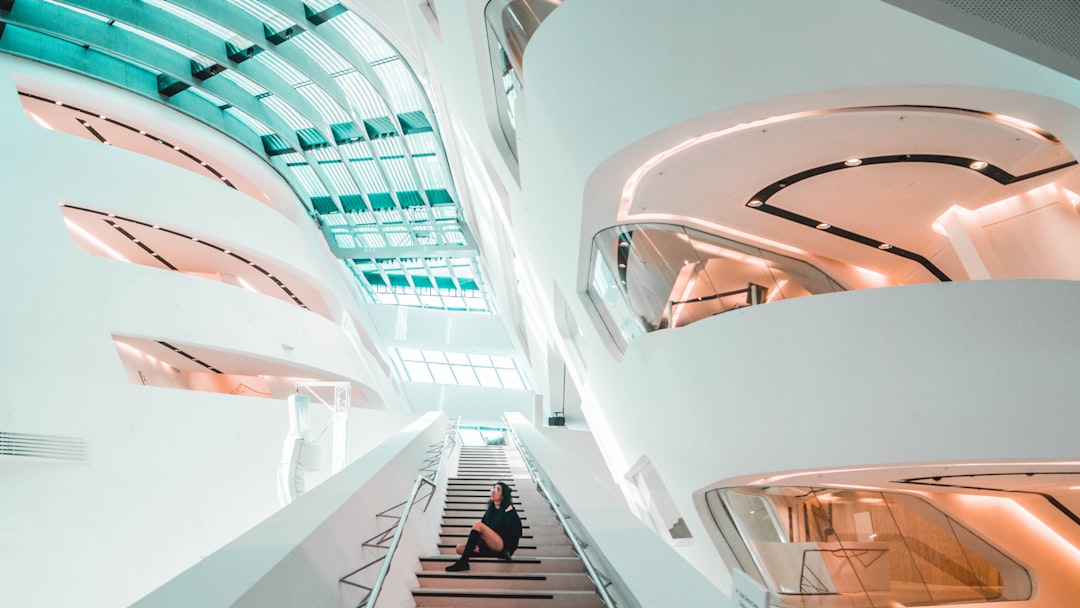
Seismic resilience is a crucial aspect of modern structural design. It's about creating building systems that can withstand the impact of earthquakes and quickly recover their functionality. The focus is on advanced systems capable of minimizing damage and facilitating rapid recovery.
Techniques like base isolation are key to achieving this. They involve strategic placement of isolation devices that act as shock absorbers, effectively reducing the transmission of seismic forces to the building's structure. This approach is increasingly integrated with advanced monitoring systems that provide real-time data on the building's condition. This allows for faster and more informed decisions during and after seismic events, contributing to efficient response and recovery.
We are also seeing the emergence of smarter materials and more sophisticated design methodologies like performance-based design. These offer exciting possibilities for future resilient systems. However, the complexities of seismic events and the diverse challenges posed by them mean continuous research and innovation are essential.
Ultimately, the focus on seismic resilience stems from the understanding of the devastating socio-economic impact of earthquakes. A commitment to innovation and continuous improvement in structural design is crucial for ensuring the safety and well-being of communities in earthquake-prone areas.
The pursuit of seismic resilience in advanced structural systems involves delving into a fascinating array of innovations, pushing the boundaries of structural engineering. We're seeing a shift away from simply preventing collapse to a focus on ensuring buildings can function effectively after an earthquake.
One exciting development is the integration of energy-dissipating devices. These dampers are like shock absorbers for buildings, soaking up the force of seismic energy and preventing it from transferring to the structure. This is a crucial aspect of minimizing damage and enhancing resilience.
Another game-changer is base isolation. This technology involves separating the building from its foundation with specialized devices that absorb earthquake motions. Imagine the building floating on a cushion during an earthquake. It's a truly ingenious approach to decoupling the structure from the ground, minimizing the destructive forces it would otherwise face.
Then there are the fascinating developments in materials. The use of hybrid materials, combining the strengths of different materials like steel and fiber-reinforced polymers, opens up a whole new world of possibilities. These structures can be simultaneously lighter and stronger, offering greater resistance to seismic forces.
The adoption of performance-based design is also very promising. Instead of focusing solely on preventing collapse, this approach sets specific performance targets for buildings during an earthquake. This shift in focus aims to ensure buildings not only survive but remain functional, allowing for swift recovery after an event.
Engineers are increasingly employing advanced computer simulations to conduct dynamic analyses of buildings. These simulations can analyze a wide range of earthquake scenarios, providing valuable insights into the anticipated performance of a structure. This level of precision allows for more informed design choices, leading to better resilience.
Smart materials are revolutionizing how we design resilient buildings. These materials can adapt to changing conditions, even during an earthquake, offering enhanced protection and valuable data about the event's effects.
It's important to acknowledge the influence of shape and geometry in seismic resilience. The design of the building itself is critical. Certain shapes and configurations are more effective at distributing seismic forces, ensuring greater structural stability.
Existing structures can be made much more resilient through retrofitting. Installing advanced seismic systems can significantly enhance their ability to withstand earthquakes, offering a more cost-effective solution than demolition and rebuilding, especially in dense urban areas.
The distribution of mass within a building can dramatically influence its response to earthquakes. By carefully strategizing mass placement or utilizing lightweight materials, we can manipulate the center of mass, enhancing overall stability.
Finally, navigating the complex landscape of global seismic design codes is crucial for achieving resilience. These codes, which vary by region based on local risk assessments, define performance expectations and building classifications. Understanding these codes is essential for making informed design decisions that comply with local requirements and prioritize safety.
Análisis Comparativo Eficiencia y Sostenibilidad de Elementos Estructurales en Edificaciones Modernas - Life Cycle Assessment of Innovative Building Components
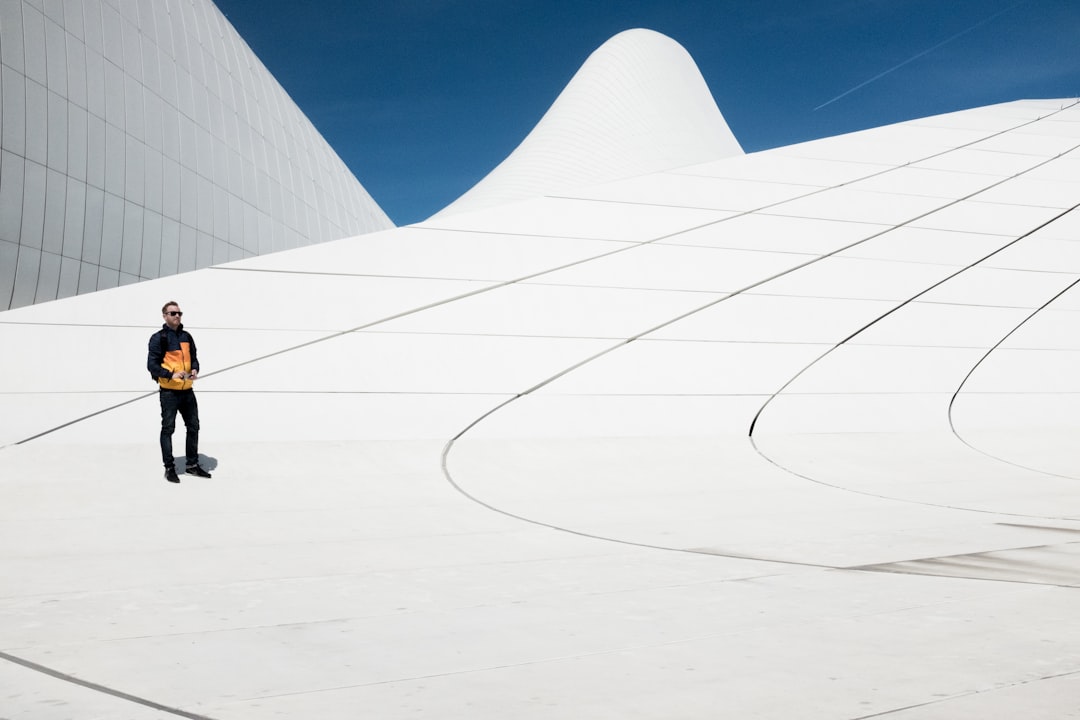
The Life Cycle Assessment (LCA) of innovative building components is a crucial step towards understanding the environmental footprint of modern construction. Since buildings are a significant source of energy consumption and greenhouse gas emissions, LCA provides a comprehensive method for evaluating materials and construction methods from cradle to grave. This assessment is particularly relevant to the development of Nearly Zero Energy Buildings (NZEBs), which prioritize energy efficiency through design and materials selection. Research has shown that advanced multifunctional building components and eco-materials can significantly reduce embodied emissions and improve thermal performance. However, while innovative technologies offer exciting potential, we need to critically examine their lifecycle impacts to ensure we are truly building sustainably.
Examining the lifecycle of innovative building components is critical to understanding their true impact on sustainability. We are seeing a fascinating range of developments, from advanced materials to cutting-edge construction techniques. For instance, modular units are generally designed for a lifespan of 30 years, while more resilient materials like engineered wood can achieve lifespans approaching 50 years with proper maintenance. This highlights the importance of choosing components with lifespans aligned with project goals.
Thermal performance is another critical factor. Prefabricated components with cutting-edge insulation materials can achieve remarkably low U-values, reaching as low as 0.10 W/m²K. This significantly improves the building's overall energy efficiency, potentially reducing heating and cooling demands.
A critical area of focus is embodied energy. While steel production is energy-intensive, its inherent durability and high recyclability mean that the embodied energy per functional year might be lower compared to some conventional materials.
Innovative components often employ non-destructive testing (NDT) methods like ultrasonic testing to assess the integrity of materials without compromising the structure. This is a crucial step towards enhancing safety and reliability during construction and throughout the building's lifespan.
Emerging technologies like 3D printing of building components are revolutionizing construction. These techniques can reduce material waste by up to 90%, representing a significant shift in how we approach material utilization during construction.
One of the most exciting developments is the emergence of self-healing materials. Self-healing concrete, for example, can autonomously repair cracks through chemical reactions, extending the lifespan of structural components.
Connection design is crucial. Innovative designs can withstand greater forces while streamlining assembly, leading to construction times up to 30% faster than traditional designs.
Smart building components are increasingly incorporating adaptive features, adjusting their insulation properties based on external temperatures to optimize energy consumption in extreme weather conditions.
Digital twin technology is playing a crucial role in optimizing building performance. It enables real-time monitoring and simulation of innovative components, providing valuable insights for predictive maintenance and improving operational efficiency throughout the building's lifecycle.
The integration of embedded sensors within structural components allows for continuous health monitoring, providing crucial data on stress and strain. This enables the prediction of potential failures before they occur, further enhancing safety and reliability.
While these innovations offer compelling possibilities, it's crucial to carefully analyze the various factors, such as material selection, manufacturing processes, and lifecycle performance, to maximize the benefits and minimize the potential downsides. The exploration of innovative building components presents both challenges and opportunities, pushing us to think critically about sustainability and future building practices.
Análisis Comparativo Eficiencia y Sostenibilidad de Elementos Estructurales en Edificaciones Modernas - Cost-Benefit Analysis of Green Building Technologies in Structures
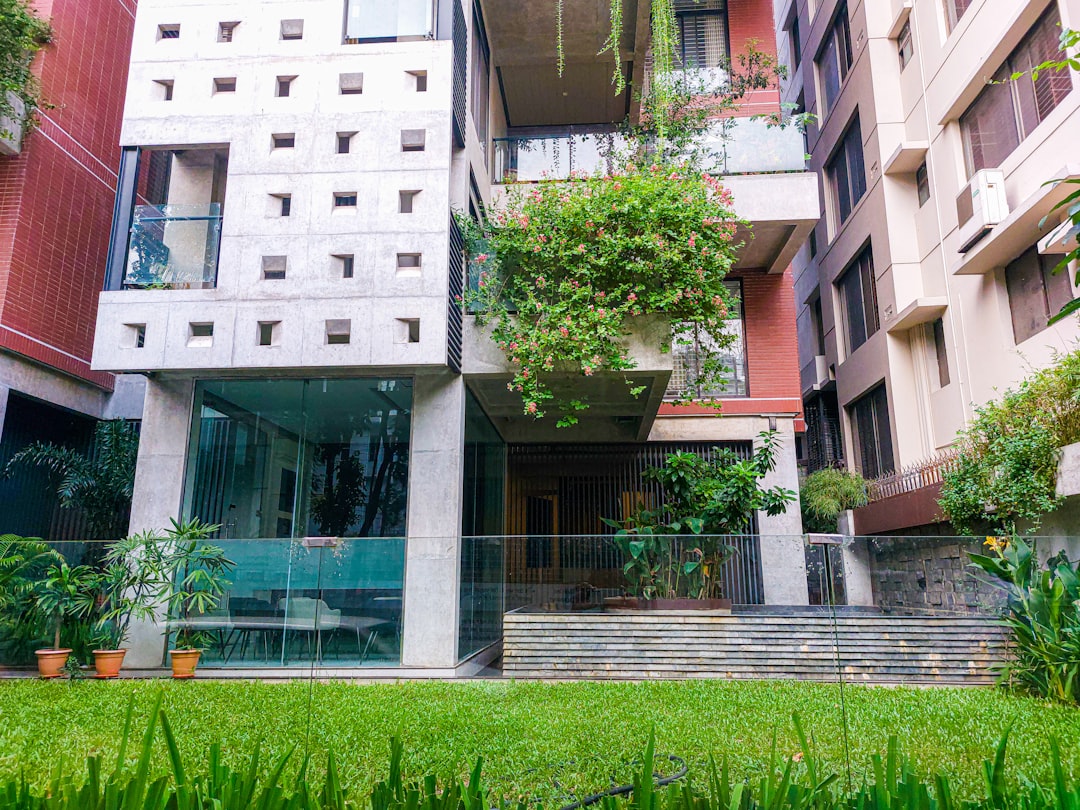
A cost-benefit analysis (CBA) is a crucial tool when evaluating green building technologies. This analysis allows for a balanced assessment of the costs incurred against the environmental and social benefits they offer. Despite the positive impact green building technologies can have on sustainability, many, such as green facades or building-integrated greenery, often come with a significant initial cost. This can be a deterrent for construction companies, especially in regions like China where green building practices are still developing.
The absence of standardized metrics for measuring the economic benefits of green buildings makes it difficult to conduct a comprehensive CBA. This lack of consistency leads to variations in the conclusions reached across different studies. Although advocates for green building emphasize the long-term advantages of these technologies, including their contributions to national sustainability goals, the fluctuating green cost premiums continue to spark debate among stakeholders. To make well-informed decisions in modern construction practices, a more detailed understanding of the financial implications and long-term effects of green building technologies is needed.
The cost-benefit analysis of green building technologies is a crucial factor in evaluating their effectiveness. While initial investments in these technologies might seem hefty, the long-term returns can be substantial. For example, implementing energy-efficient materials like phase change materials can significantly lower energy consumption, potentially saving up to 40% on heating and cooling. This, over time, compensates for the initial material costs.
Interestingly, even the orientation of a building can greatly impact energy efficiency. Strategic design, using architectural shading elements, can lead to substantial energy savings, potentially as high as 25%. However, the cost-benefit picture isn't always straightforward. Some green technologies, like high-performance insulation and triple-glazed windows, might carry higher initial costs but provide considerable long-term returns. For instance, certain high-efficiency insulation can last for more than 50 years, resulting in significantly lower operational costs in the long run.
Another crucial aspect is water efficiency. Green building technologies like rainwater harvesting and greywater recycling systems can lead to a remarkable reduction in potable water use, often by as much as 50%. This not only saves costs but also benefits the environment.
Furthermore, buildings equipped with advanced green technologies like seismic-resistant designs and flood-proof materials can be significantly more resilient against natural disasters, potentially reducing repair costs by up to 30% compared to traditional building methods. These technologies also come with a market advantage. Structures incorporating green building technologies often command higher real estate values, reflecting growing consumer interest in energy-efficient buildings.
It's worth noting that these technologies often lead to reduced maintenance costs. Materials like fiber-reinforced polymers, resistant to corrosion and damage, minimize upkeep expenses and ensure a longer lifespan for building components. Additionally, adopting hybrid energy systems that combine renewable sources like solar panels with traditional energy sources can reduce energy costs by as much as 50%, improving efficiency and reducing reliance on grid power.
It is important to recognize the impact of user behaviour on energy use. Smart building technologies like energy monitoring systems can drive behavioural changes, potentially leading to energy demand reductions of up to 20%. This underlines the importance of integrating user interaction in assessing the cost-benefit outcomes of green technologies.
The complexity of evaluating cost-benefit analysis for green building technologies requires a nuanced approach. While the initial investment may seem high, the long-term benefits in energy savings, water conservation, durability, and market appeal can significantly outweigh the initial costs, making green building a worthwhile investment in the long run.
Revolutionize structural engineering with AI-powered analysis and design. Transform blueprints into intelligent solutions in minutes. (Get started for free)
More Posts from aistructuralreview.com: