Revolutionize structural engineering with AI-powered analysis and design. Transform blueprints into intelligent solutions in minutes. (Get started for free)
Engineering Analysis Key Design Differences Between Sanitary and Storm Sewer Systems in Urban Infrastructure
Engineering Analysis Key Design Differences Between Sanitary and Storm Sewer Systems in Urban Infrastructure - Pipe Material Selection Between Stainless Steel vs HDPE Based on Flow Characteristics
Within urban sewer systems, the selection of pipe materials—specifically stainless steel versus HDPE—is crucial for ensuring efficient flow characteristics. Stainless steel, renowned for its robust nature and resistance to various environmental factors, proves beneficial in demanding environments with significant temperature changes. Its inherent strength makes it a suitable choice for applications requiring high pressure and durability. HDPE, on the other hand, presents a compelling alternative due to its flexibility, lighter weight, and inherent resistance to corrosion. These characteristics simplify installation and potentially lower maintenance needs, factors that are increasingly important in complex urban environments.
The choice between stainless steel and HDPE isn't simply a matter of material properties, however. Engineers must consider how each material impacts flow within the sewer system. Flow resistance, the tendency for a material to impede the movement of fluids, varies between the two. Additionally, the potential for clogging—a significant issue in sewer systems—can be affected by the pipe's inner surface and the material's interaction with the transported fluids. Finally, it's essential to acknowledge the unique requirements of sanitary and storm sewer systems. The type of wastewater or runoff managed within each system can influence the ideal material choice to ensure the system functions efficiently and lasts for its intended lifespan. Ultimately, a careful evaluation of these factors alongside the project's specific design criteria is essential for selecting the most appropriate pipe material for optimal sewer system performance.
When comparing stainless steel and HDPE for pipe applications, particularly within sewer systems, their flow characteristics play a crucial role in material selection. Stainless steel's inherent resistance to corrosion, especially in aggressive environments, is a major advantage. However, HDPE, though generally resistant, may be vulnerable to certain chemical interactions, limiting its use in specific situations.
The internal smoothness of stainless steel leads to a lower friction coefficient compared to HDPE. Consequently, stainless steel experiences less energy loss due to friction, potentially impacting flow rates, especially in large pipes dealing with significant flows. This difference becomes critical when designing systems to manage peak flow events.
Stainless steel exhibits a much wider temperature tolerance range than HDPE, which can lose structural integrity at relatively low temperatures. This characteristic is particularly important when considering applications involving hot wastewater or industrial discharge, where higher temperatures are expected.
The substantial weight difference between the two materials directly impacts logistics and installation. Stainless steel's heavier nature increases transportation and handling costs, as well as the necessary structural support requirements for the pipe system. Conversely, HDPE's lightweight nature makes it easier to transport and install.
HDPE's flexibility allows it to adapt to ground movement or settling without fracturing, offering a significant advantage in seismic zones or areas with unstable soil conditions. This adaptability can be crucial in minimizing damage from ground shifts.
Installation methods also differ. HDPE is frequently joined using fusion welding, enabling long continuous lengths and reducing the number of joints, streamlining the installation process. Stainless steel connections, however, often rely on flanges or welding, which can complicate and prolong the installation procedure.
The smoother internal surface of stainless steel can accommodate greater flow capacities compared to HDPE at equivalent diameters due to reduced turbulence. This factor becomes paramount in designing sewer systems capable of handling peak flows efficiently.
Stainless steel pipes generally have higher pressure ratings than HDPE. This aspect is critical in environments requiring high-pressure systems, ensuring the structural integrity of the system under extreme conditions.
The anticipated service life of stainless steel is generally longer than that of HDPE. While stainless steel can last 50 years or more in ideal conditions, HDPE's lifespan can range from 30 to 50 years depending on factors like environmental conditions and exposure.
Finally, stainless steel joints often provide a more robust and leak-resistant seal compared to HDPE connections. This distinction becomes significant in high-pressure or variable temperature applications, where maintaining joint integrity is crucial to prevent leaks. The effectiveness of the HDPE joint design is critically important.
Engineering Analysis Key Design Differences Between Sanitary and Storm Sewer Systems in Urban Infrastructure - Depth Requirements and Installation Standards for Urban Dual Systems
In urban environments, the successful implementation of dual sewer systems, encompassing both sanitary and storm sewer components, hinges on adhering to precise depth requirements and installation standards. These dual systems, often designed to handle a range of storm intensities, typically feature storm sewer conduits for minor events and overland drainage for more severe ones. This approach strives to balance efficient stormwater management with effective mitigation of urban flooding.
Proper depth and alignment are crucial to preventing issues like cross-contamination between the sanitary and storm sewer systems, a significant concern in urban settings. Furthermore, adhering to design standards ensures the systems can handle the variable flow volumes they're meant to manage, especially during peak flow events, which are increasingly important in a changing climate.
Updated guidelines stress the growing importance of factors like hydrology and hydraulics in system design. They emphasize proactive maintenance practices to bolster the long-term resilience of these systems, which are increasingly vulnerable to the effects of urbanization and climate change. A key challenge is ensuring the design considers the potential for increased stormwater runoff in the future.
The success of urban dual sewer systems depends on meticulous planning and adherence to proper installation techniques. This comprehensive approach is crucial to ensuring the functionality and sustainability of these vital infrastructure components within urban environments, allowing them to adapt and continue to serve their purpose long-term.
Urban dual systems, encompassing both storm and sanitary sewers, present a unique set of challenges when it comes to depth requirements and installation. Simply ensuring gravity flow isn't enough; engineers must also account for local groundwater conditions. High water tables can cause pipes to float if not buried deep enough, adding a layer of intricacy to design and construction.
The minimum depth for these systems isn't a universal standard. Each city often has its own rules, sometimes demanding even deeper installations than standard codes due to past flooding events. This illustrates the fragmented nature of urban infrastructure standards across different localities.
When excavating for stormwater pipes, the trench width is crucial. It depends on factors like the pipe's diameter and material. If the trench isn't correctly sized, it can weaken the pipe's structural integrity or complicate the backfilling process.
The order in which pipes are installed is often overlooked, but is vital. In certain urban environments, stormwater lines must be laid first, followed by sanitary pipes. This helps avoid major construction conflicts and minimizes disruption to other infrastructure.
While trenchless installation methods are lauded for reducing surface disruptions, they come with their own set of depth and pressure constraints that deviate from traditional open-cut methods. These limitations need to be meticulously considered during the early planning stages.
In crowded urban environments, engineers sometimes choose to install storm and sanitary pipes at varying depths within the same trench. This is not simply a space-saving strategy, but also a way to optimize flow and make maintenance easier. However, this practice isn't consistently employed across all cities.
The discrepancies in depth between sanitary and storm systems can make it tricky to locate both systems in the same area. Engineers need to think about not just the hydraulics of each system, but also whether maintenance crews will have easy access to the pipes.
Urban planning often involves adding extra installation restrictions to accommodate potential future infrastructure or utility improvements. This can impact the required depth beyond what's typically outlined in standard engineering guidelines.
When it comes to building foundations, stormwater systems are often subject to stricter setback standards, impacting depth requirements. The specific combination of soil density and load-bearing capacity can dictate the need for deeper installation compared to sanitary sewers.
Ongoing inspections throughout the installation process, especially in urban environments with varied soil types, are essential. If depth isn't precisely maintained for dual systems, it can lead to long-term issues, like sedimentation or system failure. This reinforces the need for meticulous attention to detail throughout the entire project lifecycle.
Engineering Analysis Key Design Differences Between Sanitary and Storm Sewer Systems in Urban Infrastructure - Flow Calculation Methods Using Manning Formula vs SCS Method
When comparing methods for calculating flow, like the Manning Formula and the SCS (Soil Conservation Service) method, it's essential to acknowledge their distinct purposes and how they relate to urban sewer system design. The Manning Formula is primarily used for calculating flow in open channels, considering factors like cross-section, hydraulic radius, and channel slope. It's most effective in situations where flow is heavily influenced by the physical characteristics of the channel. Conversely, the SCS method leverages the curve number approach, which estimates runoff based on land use, soil type, and antecedent moisture. This makes it a more suitable choice for scenarios involving storm runoff, particularly in larger drainage areas.
Both approaches have significant value in storm sewer design, but they tackle different facets of flow behavior. The Manning Formula excels in scenarios with stable, steady-state flow, common in open channel flows. The SCS method, on the other hand, is more adaptable to the variable flow patterns typically seen in urban settings during rainfall events. A firm grasp of these methodologies is critical for engineers to optimize urban drainage management, especially when managing the differences in flow characteristics between sanitary and storm sewer systems. It is important to note that choosing the correct method is crucial to avoid inaccurate design calculations which can lead to significant infrastructure failures and costly retrofits.
1. The Manning formula, commonly employed for calculating flow in open channels, utilizes a roughness coefficient (n) that's heavily influenced by factors like channel material and vegetation. This makes its application somewhat inconsistent as environmental conditions can greatly affect the accuracy of the result.
2. The Soil Conservation Service (SCS) method, on the other hand, relies on assigning land use and soil type classifications to estimate how much runoff will occur. This approach can lead to a wide range of outcomes depending on how these parameters are locally defined, adding another layer of complexity to urban planning and design.
3. While the Manning formula's focus is on channel geometry and slope to estimate flow velocity, the SCS method emphasizes the infiltration capacity of the soil and the potential for runoff. This difference in approach fundamentally affects how flow is predicted.
4. In urban areas, the SCS method can sometimes overestimate runoff if the influence of impervious surfaces, like roads and buildings, isn't carefully considered. This could potentially lead to storm sewer systems that are undersized for the actual flow they need to handle.
5. The Manning equation is based on empirical data which can lead to inaccuracies when applied to new or unusual situations. This highlights the need for site-specific calibrations to ensure reliability, especially in complex urban settings.
6. The SCS method utilizes a simplified approach using Curve Numbers (CN) to categorize runoff potential. While convenient, this approach doesn't fully capture the more dynamic processes that influence runoff, such as fluctuating soil moisture content, leading to possible over or under-predictions of flow volumes.
7. When choosing between the two methods, the Manning formula is typically more suitable for flow within existing channels, whereas the SCS method shines when predicting runoff from broader catchment areas. This indicates that each has distinct uses within the larger context of urban water management.
8. Incorporating locally gathered data into SCS calculations can improve accuracy, but this introduces variability based on individual interpretation and knowledge. This can make it difficult to establish standardized practices across different regions.
9. The choice between the Manning and SCS methods can greatly impact the sizing of stormwater management infrastructure. Differences in how the methods approach flow can lead to discrepancies in design that might not perform optimally during periods of high rainfall.
10. Both the Manning formula and SCS method have been criticized for their reliance on historical data which may not fully reflect current hydrological conditions, especially in urban areas undergoing rapid development. Land use patterns change over time, influencing the way water flows through a system, and historical datasets may not always be representative of this change.
Engineering Analysis Key Design Differences Between Sanitary and Storm Sewer Systems in Urban Infrastructure - Treatment Plant Integration Standards for Combined Sewer Overflow Events
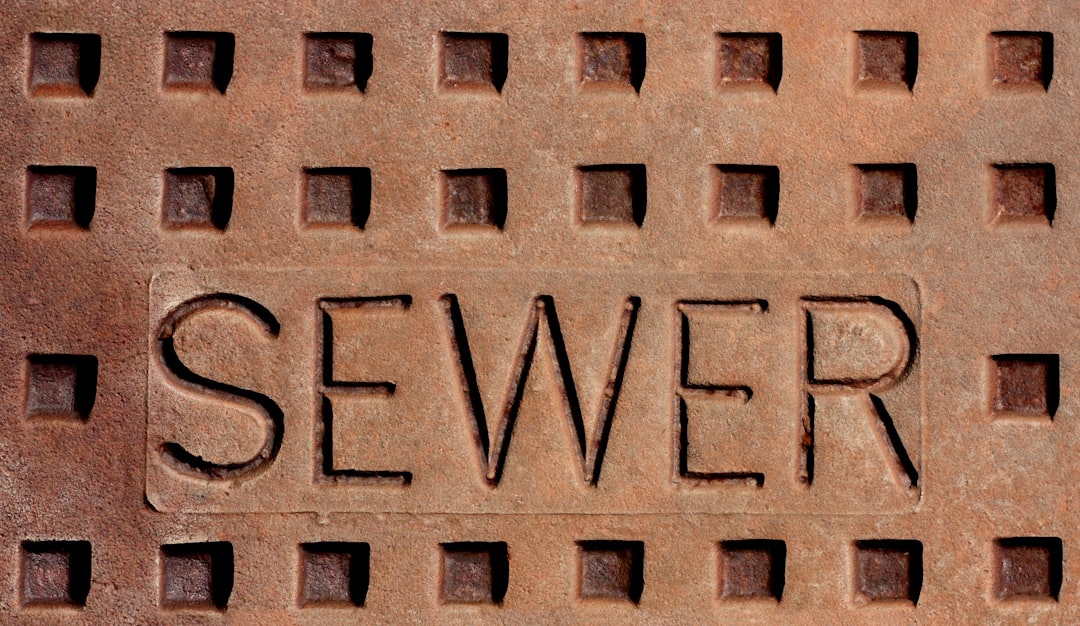
In urban areas, particularly those with established combined sewer systems (CSS), the standards for integrating treatment plant operations during Combined Sewer Overflow (CSO) events are becoming increasingly important. When heavy rainfall overwhelms the capacity of these systems, untreated wastewater and potentially harmful substances are discharged into surrounding water bodies, causing environmental degradation and jeopardizing public health. This necessitates a strong focus on developing and implementing effective CSO management strategies. These approaches range from implementing source control measures to reduce the amount of overflow, to incorporating a variety of treatment methods—from basic physical and chemical techniques to more sophisticated biological and advanced treatment technologies. Furthermore, there's a growing trend towards exploring more environmentally-friendly solutions like constructed wetlands for CSO treatment, which may offer more sustainable ways to manage urban water resources. The complexities of balancing urban infrastructure demands with environmental protection requires a deep understanding of these integration standards, especially for engineers working on CSO-related projects. The challenge, as urban environments continue to evolve, will be in effectively integrating these standards in a way that promotes both public health and environmental sustainability. While separated sanitary and storm sewer systems have been recommended to lessen the frequency of CSO events, it is important to note that implementation is not always feasible or cost-effective for existing infrastructure. As CSOs continue to present significant risks in many areas, the evolution and implementation of innovative, integrated treatment standards will be vital.
Combined sewer systems, while efficient during normal conditions, can overwhelm treatment plants during heavy rainfall, leading to Combined Sewer Overflows (CSOs). These overflows can release a significant portion of urban pollution, highlighting the need for rigorous integration standards within treatment plant design. It's becoming increasingly clear that treatment plant designs need to anticipate not just the peak flow rates associated with these events, but also the dynamic changes in sediment loads that heavily impact treatment efficiency and the quality of the final effluent.
One of the major hurdles in incorporating CSO management into existing treatment plant operations is the need for rapid responses. Treatment facilities often need to transition from their usual operating mode to a dedicated overflow management strategy within a very short time frame. This fast response requirement presents significant operational challenges.
The design and careful placement of structures like weirs and gates in CSO systems play a huge role in determining how effectively treatment plants operate. The decisions about how much overflow gets routed to treatment directly affects water quality and operating costs, so the design of these elements is crucial.
The incorporation of sophisticated flow monitoring tools like real-time hydraulic models and flow forecasting is increasing in CSO management. This reflects a shift towards data-driven design and engineering approaches, allowing for improved adaptability and efficiency during overflow events.
The highly variable nature of CSO events poses a significant challenge to treatment plants as it can result in sudden fluctuations in the quality of the incoming water. This can necessitate rapid adjustments in processes like chemical dosing and sedimentation to handle unexpected spikes in pollution levels.
To ensure that treatment standards are met even under extreme overflow conditions, treatment plant integration standards often require the incorporation of redundant treatment processes. This 'backup' approach aims to provide a safety net and ensure compliance with environmental regulations.
The distinct hydraulic characteristics of combined sewer systems demand specialized design considerations like larger storage capacities and unique treatment procedures. These features can create challenges when attempting to integrate CSO management with pre-existing treatment infrastructure.
The integration of stormwater management and treatment plants often brings with it a complex web of regulatory challenges. Engineers must carefully navigate various compliance requirements and standards which can differ significantly between different regions and jurisdictions.
Computer modeling and simulations are becoming vital tools for designing treatment plants to handle CSOs. These tools allow engineers to forecast hydraulic behavior and the expected treatment efficacy under diverse storm conditions, ensuring that the systems are robust enough to handle extreme scenarios.
The impact of CSOs on water quality is significant and the development of effective management approaches is crucial for maintaining water quality and public health. Further research into the hydraulics of combined sewer systems, and the development of improved integration standards between these systems and treatment plants is likely to become an increasingly important area of research in the coming decades.
Engineering Analysis Key Design Differences Between Sanitary and Storm Sewer Systems in Urban Infrastructure - Cross Connection Prevention Through Physical Barriers and Detection Systems
In urban water infrastructure, preventing cross-connections is crucial to protecting drinking water from contamination. This is achieved through implementing physical barriers, such as air gaps, which create a separation between the potable water supply and any potential contaminants. Backflow prevention devices also play a vital role in isolating the drinking water system from external sources. Furthermore, it's imperative to have reliable detection systems to proactively identify and address any potential cross-connection risks. The careful engineering analysis that distinguishes between sanitary and storm sewer systems is essential to this process. Effective integration of these systems minimizes the chances of contaminants entering the potable water supply, ultimately upholding the health and safety of the urban population. As urban environments grow and change, consistently adapting and improving these preventive measures is paramount for the long-term protection of both the public and the environment. While separated sanitary and storm sewer systems are beneficial for minimizing cross connections, their implementation may not always be practical, emphasizing the ongoing need for robust cross-connection prevention practices.
Cross-connections, the potential link between a clean water supply and a source of contamination, can lead to backflow, where contaminated water enters the potable water system. This risk is a constant concern in urban infrastructure, and engineers employ a variety of methods to prevent such events. Physical barriers like air gaps and backflow preventers are designed to withstand substantial pressure fluctuations, thus preventing wastewater from contaminating the drinking water supply even in extreme situations. They represent a fundamental layer of protection.
Detection systems, utilizing modern sensors, offer real-time monitoring for potential cross-connections. They leverage changes in pressure and water quality indicators to instantly alert operators about possible contamination risks. These systems are becoming increasingly sophisticated, employing machine learning to analyze historical data and potentially anticipate future events, allowing for preemptive measures.
Implementing physical barriers requires adherence to local plumbing regulations. These codes often define specific clearances and pipe types to minimize risks. The issue of cross-connections is taken seriously, and local regulations reflect this by prioritizing public health. It's interesting to see that these regulations can differ greatly between jurisdictions, indicating a local prioritization of certain aspects of cross-connection protection.
Rapid urban development can exacerbate cross-connection risks, especially where older infrastructure is located near newer systems. Comprehensive planning and assessment are crucial to mitigating potential contamination risks. As older systems age, their structural integrity decreases which, in conjunction with ever-changing landscapes, increases the risk of system failures.
Regular maintenance and testing are essential for ensuring the continued effectiveness of physical barriers. Degradation can lead to malfunctions that compromise public health, highlighting the importance of continuous monitoring and repair. As systems age, they need additional and potentially specialized maintenance and care to preserve performance.
While detection systems are advanced, they aren't foolproof. False alarms can occur due to poor calibration or sudden environmental changes, leading to operational distractions and potentially mismanaged resources. This is an interesting paradox – while designed to offer increased control, they can also pose operational risks if not properly monitored and calibrated.
The design of physical barriers often depends on the specific fluid being transported. Systems suitable for handling sanitary wastewater may not be appropriate for hazardous materials, requiring unique designs and materials. This underscores the need for engineers to carefully select barriers based on the specific conditions and risks present in a given area. The risk associated with differing fluids can greatly impact required performance parameters and subsequently affect material choice and barrier design.
The redundancy incorporated in the design of physical barriers provides a backup in case one mechanism fails. This layered approach protects the public water supply, providing a safety net if a critical component malfunctions. Redundancy can provide an added level of security by increasing the chances of a barrier continuing to prevent cross-contamination even in the event of an unexpected failure.
Integration of new cross-connection prevention systems with existing urban infrastructure can present unforeseen challenges. Engineers need to navigate spatial limitations and compatibility issues to ensure that the chosen barrier functions effectively. The addition of new systems can impact both design and execution phases of a project due to existing constraints. Engineers need to consider a variety of factors when designing and installing these systems to assure functionality and avoid creating further hazards.
In conclusion, understanding the specific threats and risks associated with cross-connections in urban settings is crucial for effective infrastructure design. Proper implementation of barriers and detection systems offers an important layer of protection for public water supplies, but maintaining and monitoring these safeguards remains critically important in an evolving urban landscape. It's evident that cross-connection control requires a thoughtful and holistic approach by engineers.
Engineering Analysis Key Design Differences Between Sanitary and Storm Sewer Systems in Urban Infrastructure - Maintenance Access Design Requirements Including Manhole Placement Strategies
Effective maintenance access is a critical aspect of both sanitary and storm sewer system design, particularly when considering the strategic placement of manholes. Sanitary sewer systems necessitate careful manhole placement, ideally maintaining a minimum 5-foot distance from residential service connections to facilitate maintenance and minimize disruption to service lines. This strategic approach is crucial for both regular inspection and repair, as well as for mitigating potential health and environmental risks stemming from sewer failures. Storm sewer systems, on the other hand, generally benefit from simpler layouts, including straight alignments between structures and, whenever possible, placement behind curbs. This approach not only promotes efficient flow but also makes routine maintenance simpler. The specific challenges and nuances of manhole placement, especially in situations like curvilinear streets where utilities may be closely spaced, must be carefully addressed to ensure the ongoing performance and manageability of the entire sewer network. Ultimately, well-planned maintenance access, including thoughtfully positioned manholes, can have a lasting impact on the resilience and longevity of urban infrastructure.
Maintaining urban sewer systems relies heavily on effective access for routine inspections and repairs. Manhole placement, often overlooked in initial design phases, significantly impacts operational efficiency down the line. Ideally, manhole locations should be carefully chosen to balance ease of access with the overall alignment of sewer assets. Failure to consider this during initial design often leads to costly and time-consuming retrofits later.
Strategic manhole placement can dramatically minimize the need for extensive excavation during maintenance events. Well-planned access points can save substantial time and resources, potentially lowering the overall cost of maintaining the sewer system over its entire lifespan. However, the materials used in the surrounding pipelines can influence inspection frequencies. For example, HDPE pipelines, with their flexible nature, may necessitate a different maintenance schedule compared to more rigid materials like cement or stainless steel.
Historical records show that poor manhole placement can increase incidents of infiltration and exfiltration. These issues not only pose environmental challenges but also contribute to higher treatment costs due to contaminated inflows. This highlights the critical importance of careful planning.
Fortunately, advancements in technology offer solutions for improved manhole design and maintenance. Remote sensing and automated monitoring systems can now be incorporated into manholes to provide real-time data on flow rates and structural integrity. This information can be used to develop more effective and targeted maintenance strategies.
Local soil conditions also play a major role in manhole design and placement. Shallower installations, for example, can be more prone to damage or blockages from sedimentation. This necessitates the need for flexible regulatory standards that accommodate unique environmental contexts.
A less obvious factor is manhole cover elevation. Excessively high covers can lead to safety issues and create accessibility problems for maintenance personnel. This warrants a deeper discussion on developing standardized height regulations that strike a balance between safety and operational needs.
Furthermore, local zoning laws can dictate specific manhole design criteria, including setbacks from buildings, impacting both engineering decisions and broader urban design considerations. These requirements can limit design options and create a complex interplay between infrastructure and urban planning.
The choice of manhole material itself influences long-term maintenance needs. While polymer composite materials offer excellent corrosion resistance, they may require specialized repair tools and techniques. This is a trade-off engineers need to consider carefully during design.
Finally, as cities increasingly integrate green infrastructure, new design considerations emerge. Engineers now face the challenge of ensuring that permeable surfaces and landscaping don't compromise the functionality of traditional sewer access points. This integration requires a thoughtful approach to maintaining both the system's performance and the desired aesthetic elements of the surrounding environment.
Revolutionize structural engineering with AI-powered analysis and design. Transform blueprints into intelligent solutions in minutes. (Get started for free)
More Posts from aistructuralreview.com: