Engineering Marvel Unveiled Critical Analysis of Hoover Dam's 660-Foot Base Structure and Its Seismic Resilience
Engineering Marvel Unveiled Critical Analysis of Hoover Dam's 660-Foot Base Structure and Its Seismic Resilience - Base Structure Design Achieves 45000 PSF Water Pressure Resistance Through 660 Foot Foundation
The Hoover Dam's base structure is a testament to engineering ingenuity, specifically engineered to resist a colossal water pressure of 45,000 PSF. This monumental feat is achieved through the 660-foot deep foundation, which acts as a crucial anchor for the entire structure, contributing significantly to its overall stability and structural integrity. This design, however, extends beyond merely withstanding immense water pressure. It is meticulously crafted with a strong emphasis on seismic resilience. The dam is designed to withstand powerful earthquakes, minimizing the potential for damage. Central to this seismic resistance is the clever incorporation of energy dissipation systems. These mechanisms act as shock absorbers, efficiently dissipating the tremendous energy generated during seismic events. The integration of such features underscores the importance of earthquake engineering principles in modern infrastructure design, which are now considered essential for ensuring the long-term safety and robustness of critical structures.
The Hoover Dam's foundation, extending an impressive 660 feet into the earth, is a testament to the ingenuity of its designers. This extraordinary depth is directly related to the dam's ability to withstand a truly remarkable 45,000 PSF of water pressure. This immense pressure, a consequence of the reservoir's depth exceeding 500 feet, places significant stress on the structure.
The foundation's design incorporates considerations for not only the hydrostatic pressure but also the potential for seismic activity and temperature variations. These factors are intertwined and can influence the foundation's integrity over its lifespan. The schist rock foundation, a type of metamorphic rock, provides a robust and stable base, a key aspect of supporting such a massive load. It's worth noting how the foundation's depth isn't merely about pressure resistance, but also engages with geological strata, contributing to the overall seismic resilience of the dam.
While concrete's compressive strength was essential in withstanding the substantial weight and pressure, achieving this was no easy feat, especially during the dam's construction in the 1930s. Engineers tackled challenges including managing rapid water flow and the risk of erosion. The curvature of the foundation is a noteworthy aspect of the design, a feature that evenly distributes the water pressure and reduces the possibility of concentrated stresses, thereby lowering the risk of failure.
The interconnectedness of structure and function is evident in the Hoover Dam. The very forces that the dam was built to withstand – the immense water pressure – are harnessed for electricity generation, a powerful example of how engineering can turn challenges into opportunities. Moreover, ongoing monitoring of the foundation's stability through sensors highlights a contemporary approach to managing monumental structures. This proactive approach speaks to the ever-evolving nature of civil engineering, where a focus on long-term safety and performance continues to drive innovations and best practices.
Engineering Marvel Unveiled Critical Analysis of Hoover Dam's 660-Foot Base Structure and Its Seismic Resilience - Seismic Protection System Uses Multiple Expansion Joints and Variable Thickness Concrete Blocks
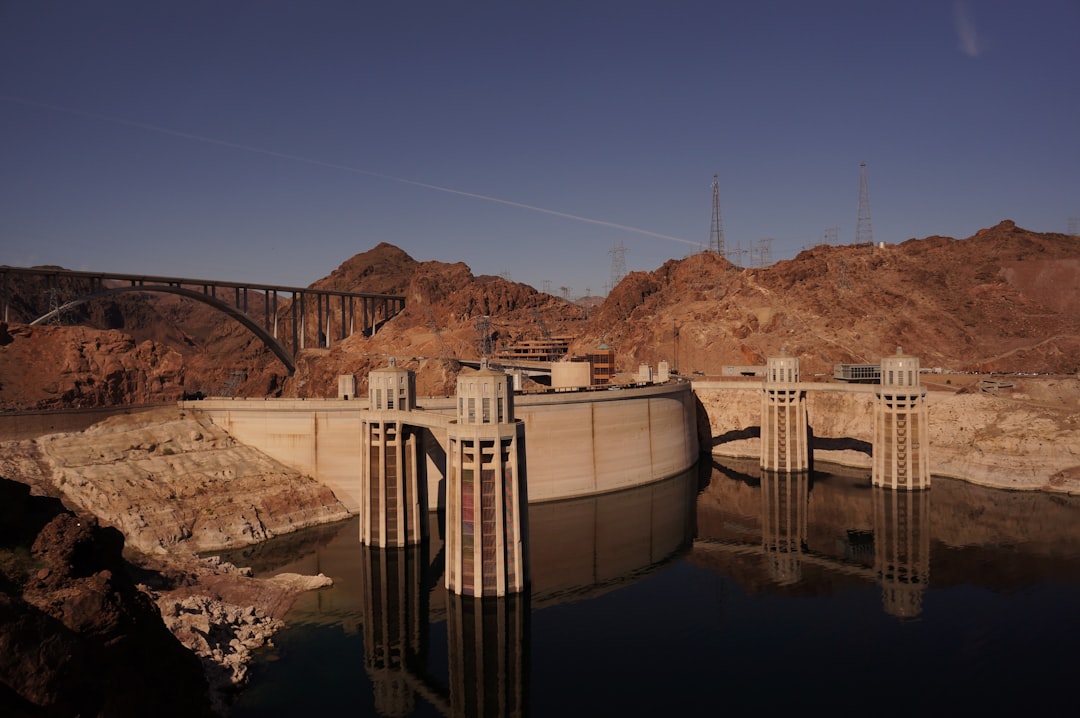
The Hoover Dam's seismic protection system features a sophisticated approach involving strategically placed expansion joints and concrete blocks of varying thickness. This design is intended to improve the dam's ability to withstand earthquake forces. The expansion joints provide flexibility within the rigid concrete structure, allowing it to accommodate the shifting and swaying that can occur during seismic activity. The use of concrete blocks with different thicknesses is another way to manage stress distribution during earthquakes, preventing concentrated forces that could lead to structural failure.
Essentially, these design features are engineered to dissipate the energy released during an earthquake. This is crucial, as it minimizes the stress experienced by the dam's foundation and overall structure. It's important to understand that the foundation, already built to withstand enormous water pressure, also needed a design to manage a different type of force – the dynamic energy from an earthquake. This showcases how earthquake engineering principles are integrated into the broader design to achieve a higher level of safety and resilience. The incorporation of these features underscores the importance of adaptability and robustness in the design of critical infrastructure in regions prone to seismic activity. It's a prime example of how engineering can anticipate and mitigate the impact of natural hazards.
The Hoover Dam's seismic protection system cleverly employs multiple expansion joints throughout its structure. These joints are crucial for accommodating the expansion and contraction that occurs due to temperature changes throughout the year. This adaptive feature allows the dam to flex and respond to stress more effectively, which is especially beneficial during earthquakes, lessening the likelihood of structural damage.
Furthermore, the use of concrete blocks with varying thicknesses demonstrates a thoughtful approach to both material usage and structural integrity. By strategically adjusting the thickness of these blocks, engineers were able to create a dam with different levels of strength and flexibility in various sections. This tailored approach helps the dam react more efficiently to the forces exerted during an earthquake, mitigating localized stress concentrations and ensuring a more uniform distribution of loads.
The expansion joints are designed to allow for movement in multiple directions. This flexibility is pivotal during seismic events as it helps the dam dissipate the energy released during earthquakes more effectively, lowering the potential for catastrophic failure. However, it's worth considering whether this design fully addresses all directions and severities of seismic forces.
Seismic events create shear stresses that can potentially destabilize the dam. The implementation of variable thickness concrete blocks helps distribute these forces more evenly throughout the structure. This evenly distributed load capacity contributes to a more robust overall response to dynamic conditions. While effective for distributing loads, we might still question how this design handles the extremes of a major earthquake.
Research into these energy dissipation systems, including the expansion joints, has involved modeling the dam's reaction to powerful earthquakes. It's interesting to see how these designs draw from both historic and contemporary earthquake-resilient design principles. However, it remains to be seen how these theoretical models hold up against the unpredictable nature of real-world seismic events.
The unique geological properties of the schist rock foundation, beyond simply providing a stable base, also influences how seismic waves travel through the ground. Understanding this geological interaction is crucial for grasping the full picture of the dam’s behavior during seismic activity. It's intriguing how the underlying rock and the dam's structure combine to create a dynamic system.
The design of the dam's base structure seems to utilize principles similar to the concept of "sacrificial elements." This means that some parts are deliberately designed to deform or fail under extreme conditions to protect more vital components of the dam from severe damage. It's a fascinating approach that prioritizes preserving the integrity of the most critical aspects of the dam. Further investigation into the interplay between the sacrificial elements and the structure is warranted.
Mathematical models used in the dam’s design involve complex simulations of earthquake behavior. These simulations show that the strategic positioning of expansion joints has a considerable impact on the dam’s overall response to various earthquake forces and their directions. It's encouraging to see this level of sophistication in the design process. Yet, the accuracy and limits of these models in predicting real-world behavior need constant scrutiny and improvement.
The construction techniques and materials used in the 1930s were cutting-edge for their time. The variable thickness of the concrete blocks not only lessened the overall weight of the structure but also enhanced its performance. This was particularly crucial, given the limitations of concrete technology available at the time. The design reflects a commitment to innovation within the limitations of the era. But, it is important to assess the long-term implications of material choices given our enhanced understanding of materials science today.
Observational studies of the Hoover Dam during past seismic events indicate that the combined use of expansion joints and variable thickness blocks is successful in reducing potentially harmful vibrations and the possibility of resonance. These are critical factors in maintaining the structural integrity and functional performance of such a massive infrastructure project. However, it's important to recognize the limitations of these observations, which may not fully capture the effects of more severe seismic events.
Engineering Marvel Unveiled Critical Analysis of Hoover Dam's 660-Foot Base Structure and Its Seismic Resilience - Gravity Arch Design Transfers Lake Mead Forces Into Black Canyon Walls
The Hoover Dam's gravity arch design is a testament to innovative engineering, skillfully directing the massive pressures from Lake Mead into the strong Black Canyon walls. This approach not only stabilizes the structure but also enhances its overall integrity. The curved shape of the dam is central to this design, as it effectively distributes the water pressure, mitigating the possibility of concentrated stress that could lead to structural failure. Moreover, the design isn't simply about passive resistance. It actively incorporates features that enhance the dam's ability to withstand earthquakes, highlighting the forward-thinking integration of seismic principles alongside the management of immense water pressure. The Hoover Dam stands as a powerful illustration of adaptive engineering, a structure designed to accommodate and respond to significant natural forces while serving as a landmark achievement of human innovation. Yet, it's crucial that ongoing assessments and studies continue to ensure the long-term efficacy of this ingenious design in the face of potential future seismic or other natural events.
The Hoover Dam's gravity arch design represents a clever solution for managing the immense forces exerted by Lake Mead. Concrete, with its superior compressive strength, acts as the primary load-bearing material, efficiently transferring the weight of the water directly into the strong Black Canyon walls. This transfer of force creates a structural equilibrium, enhancing the dam's durability and promoting a more uniform distribution of stress, thereby reducing the risk of localized failures under extreme pressure and potential seismic activity.
The arch shape itself is a notable element of this design, effectively minimizing the overturning moments created by the horizontal component of the water's pressure. This interaction between geometry and force management is a hallmark of sophisticated civil engineering projects. Black Canyon's geological features, particularly the presence of schist rock, provide a stable foundation and minimize deformation during stress events. This emphasizes how careful site selection played a critical role alongside the engineering design in ensuring the dam's long-term stability.
The Hoover Dam's design skillfully combines arch and gravity principles, maximizing vertical load support while simultaneously channeling seismic forces efficiently. This approach, which tackles multi-directional forces that can threaten structural integrity, sets it apart from simpler dam designs. It suggests a level of engineering foresight that went into carefully calculating the various types of forces the structure would endure. The design calculations weren't limited to static pressure considerations; dynamic forces associated with potential seismic events were meticulously simulated and accounted for.
The interaction between the gravity arch, the dam's structure, and the canyon walls is a key component of the dam's stability. This intricate interplay not only counters the immense gravitational forces but also effectively mitigates the dynamic loading that might occur during seismic activity. It’s interesting to note how principles incorporated into the Hoover Dam's design have parallels with modern earthquake-resistant structures. This highlights the enduring relevance of classic engineering solutions when paired with more advanced computational methods.
While the Hoover Dam's design is impressive, ongoing research continues to refine our understanding of potential future seismic threats. This highlights the importance of constant adaptation and evolution even for seemingly robust structures. As new geological data and advancements in engineering analysis emerge, the design considerations for dams and similar structures must also evolve. The "gravity arch" concept is more than just a structural feature—it embodies a powerful solution to the historical challenges of dam construction. It showcases how early 20th-century engineering principles retain their ingenuity and relevance today as engineers navigate increasingly complex environmental and structural constraints.
Engineering Marvel Unveiled Critical Analysis of Hoover Dam's 660-Foot Base Structure and Its Seismic Resilience - Internal Cooling System Maintains 39 Degree Fahrenheit Core Temperature Since 1935
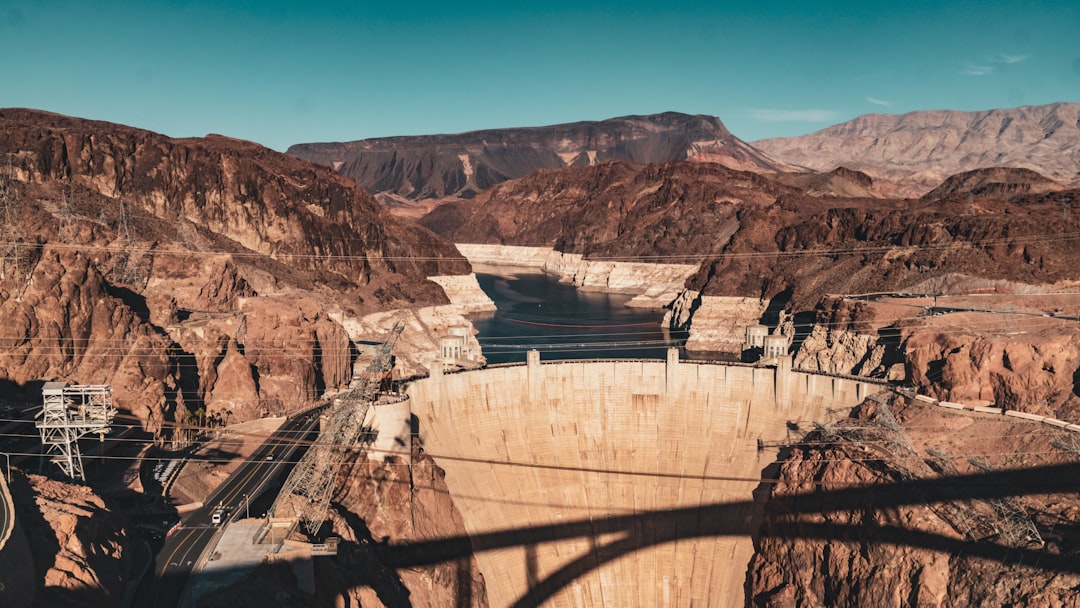
Since its completion in 1935, the Hoover Dam has relied on an internal cooling system to maintain a constant core temperature of 39 degrees Fahrenheit. This system is crucial for protecting the dam's sensitive components and materials from the damaging effects of heat. Its continued operation for nearly a century emphasizes the forethought and planning that went into the dam's design, ensuring its long-term stability. While the system was undeniably innovative for its time, it also highlights the importance of thermal management in large-scale infrastructure projects. Considering the changing climate and increased reliance on such infrastructure, questions arise about how such systems can be designed and refined in the future. Maintaining a consistent core temperature is vital not only for protecting internal structures and machinery, but it could potentially extend the life of the concrete structure and its overall resilience to environmental variations. Though the design was groundbreaking in 1935, it remains to be seen if these cooling systems are fully optimized for the future conditions they may have to withstand.
The Hoover Dam's internal cooling system, operational since its completion in 1935, has consistently maintained a core temperature of 39 degrees Fahrenheit. This is a remarkable achievement, particularly when considering the extreme temperature variations the dam experiences in the desert environment. The consistent core temperature is crucial, not just for the comfort of personnel working within the structure, but also for preserving the functionality of the complex mechanical and electrical equipment housed within the dam. This constant temperature control minimizes the risk of equipment damage caused by fluctuations in heat and cold.
The cooling system is notable for its reliance on water as a natural refrigerant, avoiding the use of potentially hazardous synthetic alternatives. This choice not only reduces the risk of harmful leaks but also likely contributes to the long-term reliability and durability of the system. It showcases a pioneering approach to resource management and environmental consideration within the field of engineering. The system operates by using gravity to circulate cool water, minimizing the need for energy-intensive pumping systems. This design choice highlights the engineers' awareness of energy efficiency and simplicity in a large-scale project.
Interestingly, the cooling system is also integrated into the overall energy management of the dam. It plays a part in dissipating heat generated by the power-generating turbines, preventing overheating and contributing to the overall efficiency of the electrical generation process. The design of the cooling system is a product of the 1930s understanding of thermodynamics and materials science. It represents a significant accomplishment of early 20th-century engineering, demonstrating foresight in anticipating long-term operational challenges related to temperature control.
The engineers optimized heat transfer by incorporating a complex network of pipes to circulate cool water through areas of the dam that generate significant heat. This focused approach ensures that every vital component remains adequately cooled, contributing to the longevity of the structure's operational life. Furthermore, the choice of building materials was carefully considered to include those with insulation properties, minimizing the amount of energy required to maintain a stable internal temperature. The system's design incorporates advanced sensor technology for monitoring temperature variations, enabling the implementation of proactive maintenance protocols. This approach allows for early detection and prevention of issues before they escalate into more serious problems, showcasing a sophisticated awareness of the need for constant monitoring in such a large and complex structure.
The choice of maintaining a 39-degree Fahrenheit core temperature likely stems from the understanding of the unique properties of water. At this temperature, water's density is optimal, allowing it to more effectively absorb and transfer thermal energy. This enhanced cooling capacity increases the overall effectiveness of the system. Notably, the cooling system is carefully integrated into the dam's structural design. This approach ensures that the stresses introduced by the cooling process do not negatively impact the structural integrity of the dam. The attention paid to integrating the cooling system into the larger structural picture exemplifies a truly holistic design approach.
Engineering Marvel Unveiled Critical Analysis of Hoover Dam's 660-Foot Base Structure and Its Seismic Resilience - Automated Structural Health Monitoring Detects 01 Inch Movement Variations
The Hoover Dam's scale and importance necessitate continuous monitoring to ensure its long-term stability. Automated systems for structural health monitoring (SHM) are crucial in this effort, allowing for the detection of even subtle movement changes, like variations as small as a tenth of an inch. These systems leverage a network of strategically placed sensors that constantly gather data on the dam's condition. This data can help engineers promptly detect anomalies that could signify developing structural issues, potentially preventing problems before they escalate into safety hazards.
Beyond immediate risk assessment, SHM contributes significantly to the dam's overall resilience, particularly in a region susceptible to earthquakes. The long-term accumulation of data provides a detailed picture of the structure's behavior and responses to various stressors. Integrating advanced analytical methods, such as AI-based algorithms, can further enhance SHM's predictive capabilities. These techniques can forecast potential future problems and optimize maintenance strategies, extending the dam's functional lifespan.
In an era of evolving environmental conditions and aging infrastructure, SHM technologies become increasingly vital. The capacity to proactively monitor and assess critical structures like the Hoover Dam helps engineers mitigate potential failures and safeguards both the infrastructure and public safety. This proactive approach underscores the importance of integrating modern monitoring practices with the core engineering design principles that have made these monumental projects possible.
The Hoover Dam's structural health monitoring incorporates sophisticated automated systems capable of detecting remarkably small movements, as little as 0.1 inch. This high level of precision in monitoring is a testament to the advanced technological capabilities employed to ensure the ongoing safety and structural integrity of the dam. These systems rely on an extensive network of sensors strategically placed throughout the structure, enabling a continuous stream of data that captures the dam's response to various environmental and operational conditions. The constant flow of data provides engineers with a detailed understanding of the dam's performance, highlighting any potential anomalies or deviations from expected behavior. Notably, these sensor systems are designed to trigger immediate alerts if any significant movement is detected. This rapid response capability empowers engineers to take proactive steps, performing maintenance or initiating repairs before minor issues evolve into larger structural problems. This approach emphasizes the crucial role of a proactive maintenance strategy for such a complex and vital infrastructure project.
Furthermore, the information collected from the sensor network is incorporated into ongoing evaluations of the dam's design and operational protocols. By continuously analyzing data on stress patterns and movement, engineers can refine and adjust the dam's operational procedures in real-time. This ability to adapt based on observed conditions is crucial, particularly when considering the impact of external forces, such as seismic events, which can induce sudden and unpredictable shifts in stress distribution throughout the dam's structure. It's noteworthy how the utilization of automated monitoring systems represents a significant departure from the engineering practices employed during the dam's original construction in the 1930s. This highlights the remarkable advancements in civil engineering that have revolutionized the methods for managing complex infrastructure projects over the past century. Moreover, the monitoring system doesn't operate in isolation. It also considers the geological environment surrounding the dam. Subtle shifts in the ground, either due to natural processes or human activity, can have a tangible impact on the dam's stability. Therefore, understanding the complex interplay between the dam's structure and the local geology is essential for comprehensive monitoring and risk assessment.
The transition to automated monitoring has greatly reduced the potential for human error in assessing the dam's structural health. The near-constant stream of precise data eliminates the possibility of missed anomalies or inaccurate interpretations that could arise from manual inspections. This aspect is essential in ensuring the utmost accuracy and timeliness in assessing the dam's integrity, thereby reinforcing public confidence in the structure's safety. Additionally, the enduring capabilities of the monitoring systems, designed to withstand the harsh desert environment for many years to come, underscore the foresight of the engineers who integrated this technology. The continued successful operation of these systems provides invaluable insights for future design considerations and strengthens the scientific understanding of managing similar structures. The data accumulated over time from these monitoring systems is not merely beneficial for short-term maintenance but also contributes to a wider understanding of long-term structural behavior. This knowledge base, accrued through continued research and data analysis, has the potential to enhance the designs of future dams and improve current engineering best practices, contributing to the ongoing advancement and innovation within the field of civil engineering.
Engineering Marvel Unveiled Critical Analysis of Hoover Dam's 660-Foot Base Structure and Its Seismic Resilience - Foundation Rock Treatment Required 422 Miles of Grout Injection to Prevent Water Seepage
The Hoover Dam's massive base, extending 660 feet into the Black Canyon, required extensive treatment to ensure its long-term stability. A key component of this was the injection of an astounding 422 miles of grout into the foundation rock. This extensive grouting was vital to prevent water from seeping into the dam's base, which could weaken the structure and undermine its integrity over time.
The grout, a mixture of cement and water, was pumped into the cracks and voids within the rock, effectively sealing them and preventing water from infiltrating below the dam. This process, also known as permeation grouting, essentially improved the overall strength and stability of the foundation. Furthermore, grout curtains were strategically placed under the dam, acting as a barrier to further reduce water seepage and control the pressure exerted by groundwater. These techniques significantly decrease the hydraulic conductivity of the rock, meaning water flows through it less easily, contributing to the overall resilience of the dam's foundation.
These measures highlight the challenges of constructing a massive infrastructure project like the Hoover Dam, where the interaction of water, rock, and the structure itself needed to be meticulously managed. It emphasizes the critical role of meticulous planning and innovative engineering solutions to address the complexities of geological and environmental factors to ensure the longevity of important infrastructure. Without proper consideration of these elements, the dam's lifespan and stability could be compromised, highlighting the enduring importance of robust engineering practices in protecting crucial structures from the forces of nature.
The Hoover Dam's foundation, extending 660 feet deep, needed extensive treatment to ensure its long-term stability. A crucial part of this treatment involved injecting an impressive 422 miles of grout into the underlying rock. This massive undertaking aimed to address the ever-present threat of water seeping into the foundation, a risk that could compromise the entire structure's integrity. This grout injection effectively changes the hydraulic characteristics of the ground around the dam, essentially acting as a barrier against water movement. It's akin to creating a "grout curtain" that prevents water from easily passing through the rock mass and into the dam's base, bolstering the foundation's resistance to the enormous water pressure from the reservoir.
The grouting process itself was a carefully orchestrated engineering feat. Engineers opted for high-strength cement-based grout, which not only fills the gaps and spaces within the rock but also strengthens the connections between individual rock pieces. This reinforcement plays a crucial role in providing the foundation with increased resilience against seismic activity, safeguarding it from the vibrations that earthquakes produce. However, the grout injection process needed precise control over the pressure used. Too much pressure could have potentially destabilized the surrounding rock, highlighting the need for advanced engineering methods and constant monitoring during this phase of construction.
Beyond simple void filling, the grout injection strategy considered the complexities of fluid flow. Engineers utilized principles of fluid dynamics to ensure the grout spread evenly and permeated the porous rock in an optimal way. The efficacy of this grout distribution is linked to both the ability to manage water seepage and to how the foundation responds to seismic forces. It's noteworthy that water seepage is considered one of the primary ways dams can fail, and this targeted grout approach is designed to address this issue. Moreover, by creating a stronger, more stable base, the foundation becomes better prepared to withstand the powerful, dynamic forces introduced by earthquakes.
The sheer amount of grout used, 422 miles, speaks volumes about the dam's anticipated longevity and structural robustness. It suggests a forward-thinking approach to address not only present-day geotechnical issues but also potential long-term challenges associated with aging infrastructure. Such a large-scale grouting treatment isn't a common practice in contemporary civil engineering, especially not at this scale. The Hoover Dam exemplifies how historical engineering solutions can be adapted to achieve exceptional levels of modern structural resilience.
Interestingly, the interaction between the injected grout and the surrounding geology influences how seismic waves travel through the ground. Understanding how these waves interact with the modified rock around the foundation is critical to understanding the dam's dynamic behavior during seismic events. Furthermore, the development of improved grout materials and application techniques since the dam's construction offers the opportunity to re-evaluate similar existing infrastructure and consider incorporating modern innovations. This continuous advancement in materials and techniques opens avenues for increasing the safety and durability of aging structures. By exploring these advancements, future engineers can potentially enhance the performance and lifespan of critical infrastructure.
More Posts from aistructuralreview.com: