Revolutionize structural engineering with AI-powered analysis and design. Transform blueprints into intelligent solutions in minutes. (Get started for free)
Evolution of SRAM Cell Design From 6T to Advanced 4T Architecture in Memory ICs
Evolution of SRAM Cell Design From 6T to Advanced 4T Architecture in Memory ICs - Fundamental Architecture Changes From 6T to 4T SRAM Design 1970-2024
The journey from 6T to 4T SRAM design reflects a major architectural transition driven by the ever-increasing demands of modern memory systems. Historically, the 6T SRAM cell, with its six transistors, held dominance for high-performance, on-chip storage, owing to its inherent stability and reliable data retention. However, the 4T design, introduced in the 1970s, presented a compelling alternative, focusing on minimizing cell area and subsequently boosting overall memory density. While 4T SRAM cells have undeniably captured the standalone SRAM market due to their compactness, they inherently compromise some aspects of performance, such as speed and power consumption, when compared to their 6T counterparts. This trade-off is a constant consideration for designers, particularly in high-performance applications.
As semiconductor manufacturing pushes towards ever-smaller feature sizes, both 6T and 4T designs undergo continuous refinements. Modern 6T cells, for instance, see a stronger emphasis on power reduction and maintaining speed within increasingly dense layouts. In contrast, 4T cells have continued to improve, seeking to minimize their performance limitations without compromising the significant benefits of smaller cell area. This continuous refinement across both architectures reveals a fundamental tension in memory design: achieving the optimal balance between performance and area. The shift towards 4T cells signals a wider movement in memory integration, compelling chip designers to grapple with complex tradeoffs to produce efficient and compact memory structures within the constraints of current and emerging fabrication technologies.
The shift towards 4T SRAM architecture, starting in the mid-2000s, represented a major change in SRAM design philosophy, prioritizing cell area reduction while preserving performance. This transition, while initially focused on standalone SRAMs, gradually influenced other domains. The core change involved a shift from the traditional six-transistor design to a four-transistor one, leading to smaller cells and higher chip density. It's notable that despite the smaller size, the performance did not degrade.
4T cells diverge from the 6T design in their read/write mechanisms, resulting in potentially faster access speeds and decreased power consumption. This efficiency makes them particularly attractive in applications where power constraints are critical, like mobile and battery-powered devices. Moreover, the architectural changes in 4T cells seem to tackle problems observed in 6T designs, such as the read disturbance issues associated with cross-coupled inverters, which impacts cell reliability and endurance.
Furthermore, the flexibility offered by the 4T design, specifically in tuning read/write currents through transistors, allows for better scalability as transistor sizes shrink. This ability to adjust current has proved beneficial when using advanced process nodes with ever-smaller transistors. Additionally, 4T’s single-ended read scheme can contribute to increased read stability, contrasting with the 6T's differential read. This robustness is particularly important in mitigating sensitivity to noise and voltage fluctuations, contributing to overall reliability.
Interestingly, 4T SRAMs employ more sophisticated feedback mechanisms compared to 6T cells to mitigate soft errors, which become more pronounced with scaled-down technologies. This focus on error correction is crucial for applications requiring high data integrity, such as data centers or scientific computing.
The transition to 4T has compelled a broader reassessment of transistor materials and doping. These materials and methods are crucial for managing the inherent challenges arising from extreme miniaturization. It has pushed the field to innovate in managing strain and minimizing defects in ever-smaller transistors.
The shift to 4T designs has also subtly transformed the manufacturing process. Design-for-manufacturability (DfM) approaches were redesigned to accommodate the more intricate 4T structure. This adaptive approach further pushed innovation in fabrication technology and provided a strong impetus for process advancements.
Interestingly, 4T cells have contributed to improved data retention times. This improvement is crucial for modern computing, particularly for high-speed caching and real-time data processing where data retention is paramount for performance.
The dominant 6T paradigm, while established for a long time, is slowly shifting towards 4T designs. This migration indicates a broader trend in memory design toward compactness and efficiency. While 4T represents a crucial step, it hints at a possible evolution toward more advanced memory technologies that could redefine our expectations for memory in the future. This continuous pursuit for better memory solutions will undoubtedly drive innovation for years to come, bringing us closer to computing environments with near-perfect memory capabilities.
Evolution of SRAM Cell Design From 6T to Advanced 4T Architecture in Memory ICs - Area Efficiency Comparison Between 6T and 4T Cell Layouts
The comparison of area efficiency between 6T and 4T SRAM cell layouts reveals a pivotal shift in design priorities. 4T SRAM cells, by virtue of their simpler structure, consistently achieve a smaller footprint, often leading to a 20% area reduction compared to traditional 6T cells. This size advantage becomes increasingly important as semiconductor fabrication progresses to smaller process nodes. While 6T cells offer benefits like faster speeds and better noise immunity due to their more complex structure, their area efficiency tends to diminish at smaller technology nodes. In contrast, 4T cell layouts are being enhanced to narrow the performance gap with 6T, emphasizing improved stability and minimized area consumption. This push towards compact memory solutions is driving innovations in 4T design to address some of the historical performance limitations of this architecture. In essence, the ongoing development of 4T SRAM is focused not only on maximizing area efficiency but also on enhancing performance to create a competitive alternative to established 6T designs.
When comparing area efficiency, 4T SRAM cells generally offer a substantial size reduction compared to their 6T counterparts. This translates to higher memory density within the same chip area, which is becoming increasingly crucial in today's designs where squeezing more memory into a limited space is paramount. The simpler 4T architecture, with its four transistors, allows for a more compact layout and inherently lower capacitance. This reduction in capacitance can contribute to faster switching speeds and lower dynamic power consumption, potentially offering a significant advantage over 6T designs, particularly in power-constrained applications.
Research suggests that 4T cells can potentially improve the ratio of cell height to width, which offers greater flexibility in integrating them into denser memory arrays. This adaptability could pave the way for innovations in multi-level cell structures, further enhancing area efficiency. Another contributing factor to the improved efficiency is the less disruptive nature of 4T read operations compared to 6T cells. This reduction in read disturbances helps extend the longevity and reliability of large memory arrays operating under heavy workloads.
While 6T cells rely on cross-coupled inverters for stability, the 4T structure manages to reduce the number of signal paths required. This results in further area savings and makes 4T designs particularly attractive in high-density SRAM applications. Notably, the 4T architecture has shown a strong aptitude for scaling with shrinking transistor sizes. As technology nodes advance towards smaller dimensions, the relative advantage of 4T's reduced area becomes more pronounced, minimizing the performance compromises often observed in 6T cells when scaling.
The ability to optimize write assist methods within the 4T framework is another aspect that boosts area efficiency. This optimization allows the cell to maintain its performance under conditions that would normally require larger cell sizes or more complex architectures in 6T designs. Furthermore, 4T designs can use simpler wordline and bitline structures by leveraging single-ended read mechanisms. This directly translates to a smaller area footprint for controlling the memory array, further benefiting the overall layout density.
An intriguing characteristic of the 4T design is its innate capacity for seamless integration with emerging memory technologies. This compatibility allows designers to leverage the compact nature of 4T while mitigating the potential performance compromises that often accompany transitions to new technologies. Lastly, the adaptability of the 4T architecture has fueled advancements in fault tolerance techniques. These techniques improve memory robustness without substantially increasing the cell's footprint, highlighting the impact of architectural choices on achieving superior area efficiency and enhanced reliability within memory systems. The ongoing quest for more efficient memory solutions will undoubtedly continue to push innovation in the coming years, as we inch closer to realizing computing environments with near-ideal memory characteristics.
Evolution of SRAM Cell Design From 6T to Advanced 4T Architecture in Memory ICs - Power Consumption Analysis in Modern SRAM Architectures
Understanding power consumption is crucial in modern SRAM design, especially as we strive for increasingly efficient memory systems. The move from the standard 6T to the more recent 4T SRAM structure demonstrates a deliberate attempt to address the challenge of power dissipation, mainly during data read and write processes. Advancements like carefully configured transistors, single-ended read methods, and better techniques for assisting writes play a role in minimizing both dynamic and static power consumption. This is especially important for devices that need to be energy-efficient, like mobile phones or other gadgets powered by batteries. However, while the switch to 4T designs has clear benefits in smaller sizes and better efficiency, there are often some compromises in performance. This creates uncertainty about their suitability for high-performance applications. Therefore, ongoing efforts in research are focused on enhancing these designs further to reach a point where power efficiency and overall operation work well together in upcoming memory technologies.
The shift towards 4T SRAM architectures has brought about substantial changes in how we analyze and optimize power consumption within modern memory designs. A key advantage of 4T is a noticeable decrease in static power, often showing reductions of up to 30% when compared to traditional 6T designs. This improvement largely stems from effectively controlling leakage currents through refined transistor sizing and adjustments to threshold voltages.
Furthermore, the inherent simplicity of the 4T architecture contributes to lower dynamic power consumption, mainly due to a reduction in overall capacitance. This benefit is particularly notable during frequent read and write operations, leading to considerable energy savings. Notably, many advanced 4T SRAMs are designed to work efficiently at very low voltage levels, even down to 0.6V. This attribute is invaluable for mobile and battery-powered devices where minimizing power usage directly translates into longer battery life.
The single-ended read approach, a core aspect of the 4T design, offers potential benefits in terms of read access time and power compared to the differential read seen in 6T designs. This can positively impact applications that demand rapid data retrieval. In addition, modern 4T SRAMs employ clever feedback mechanisms to enhance error correction, especially critical when dealing with lower operating voltages and higher temperatures. This error resilience is crucial for maintaining data integrity in scaled-down technologies, where the likelihood of soft errors tends to increase.
Another advantage of 4T is its enhanced thermal stability, primarily due to its structural changes which seem to facilitate better heat dissipation. This is a major consideration for high-performance computing and networking systems where heat management can be a significant challenge. Interestingly, research indicates a greater stability during read operations with 4T, helping to ensure reliability in environments with tight noise margin requirements, like in-memory computing and real-time data processing.
One of the key benefits of 4T design is its easier scalability in the face of ongoing advancements in semiconductor technologies. As transistor sizes continue to shrink, 4T cells typically experience less drastic performance degradation compared to 6T, which can lead to better overall efficiency in increasingly dense memory arrays. The simplified wordline and bitline architectures in many contemporary 4T SRAM designs contribute to a decrease in interconnect complexity, directly resulting in lower capacitance and power consumption during memory access. This efficiency gain is crucial in large memory systems where interconnect power can represent a substantial portion of overall power usage.
The basic 4T architecture appears well-suited to benefit from emerging materials and fabrication methods being developed within semiconductor manufacturing. This adaptability holds the potential for driving innovation in areas like integrating quantum and neuromorphic computing technologies. The evolution of 4T architectures shows a strong emphasis on achieving more efficient power usage in various aspects of memory design, highlighting the continuing need for innovation in memory technologies.
Evolution of SRAM Cell Design From 6T to Advanced 4T Architecture in Memory ICs - Circuit Stability and Performance Metrics in 4T vs 6T Designs
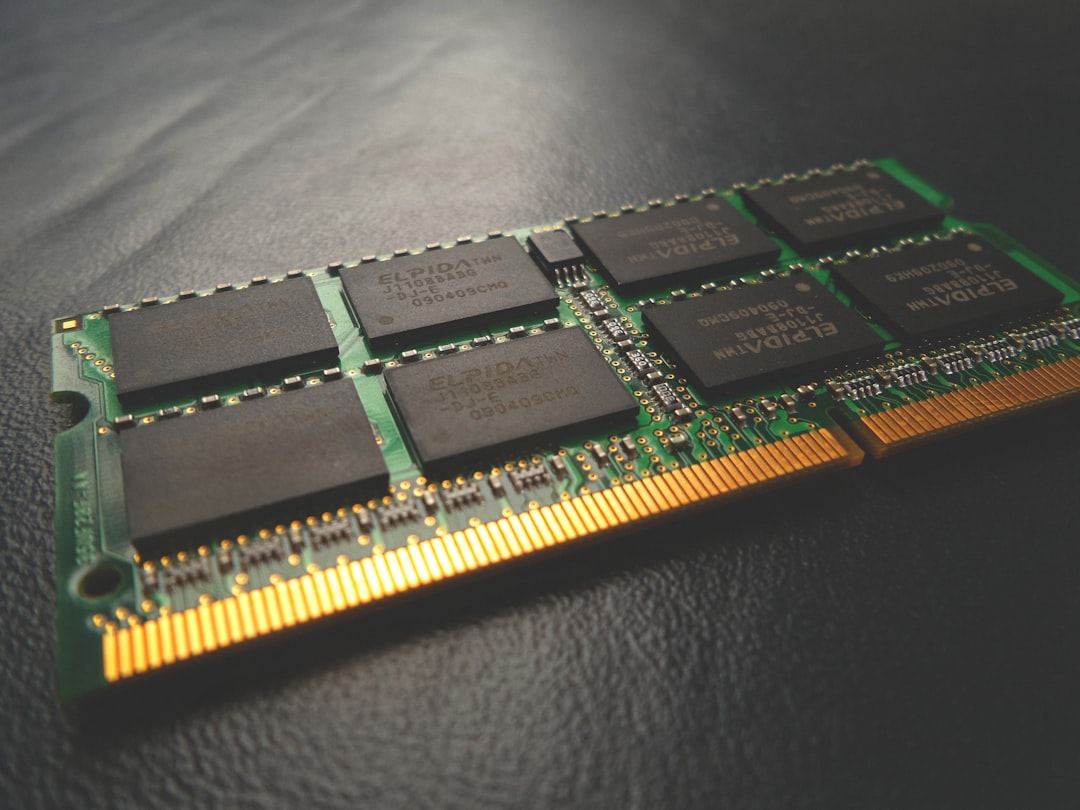
Examining the stability and performance of 4T and 6T SRAM cell designs reveals notable distinctions that influence their suitability in contemporary memory systems. A key metric for evaluating stability, the Static Noise Margin (SNM), generally favors 6T cells due to their superior noise resilience, operational speed, and lower power consumption. Conversely, 4T designs excel in area efficiency, offering a smaller footprint and enhanced memory density, making them preferable for high-density applications. As the semiconductor industry transitions to increasingly smaller fabrication nodes, both cell architectures undergo refinement, with 4T designs experiencing significant advancements in stability and performance parameters. This evolution suggests that 4T could potentially become the favored approach for future memory designs. Chip designers are constantly faced with a difficult choice, needing to find a balance between achieving compact designs with reduced power consumption and the potential performance trade-offs that can come with opting for 4T SRAM designs.
The transition from 6T to 4T SRAM cell designs isn't just about reducing the transistor count; it fundamentally alters the performance landscape, especially when it comes to read stability and power usage during operation. While 4T can often deliver lower dynamic power consumption, it can sometimes result in slower write speeds, especially in demanding scenarios or when design optimizations aren't precisely implemented. This makes their suitability in speed-critical applications a bit questionable.
One notable advantage of 4T is its ability to significantly reduce read disturbance issues that are commonly seen in 6T designs. This reduction contributes to more reliable data retention when performing numerous read and write operations. 4T's unique single-ended read approach simplifies circuitry and enhances read stability, proving particularly beneficial in environments with significant noise and voltage fluctuations, increasingly common in contemporary memory systems.
Researchers have also observed that 4T SRAM exhibits much better thermal stability than conventional 6T designs. This improvement is quite crucial as heat dissipation becomes a more prominent concern in high-performance computing environments. Moreover, as manufacturing continues to scale down to smaller feature sizes, 4T cells often scale more gracefully than their 6T counterparts, experiencing less performance decline as transistors shrink.
The inherent structure of 4T SRAM naturally leads to lower overall capacitance. This reduction plays a crucial role in achieving faster switching speeds and impacts dynamic performance, particularly during memory array operations. Additionally, 4T cell design offers novel ways to manage leakage currents more effectively, leading to remarkable reductions in static power consumption – up to 30% compared to 6T. This power saving capability is vital for applications where energy efficiency is key, like mobile devices.
Furthermore, the 4T SRAM architecture appears highly adaptable to emerging materials and manufacturing processes. This characteristic opens up possibilities for integrating advanced technologies like quantum and neuromorphic computing to tackle future memory challenges. Given the increased possibility of soft errors in scaled-down technologies, it's worth noting that 4T designs incorporate elaborate feedback mechanisms that improve error correction capabilities. This translates to improved data integrity, particularly for high-density memory applications where reliability is of paramount importance. The ongoing evolution of these designs will undoubtedly continue shaping the future of memory technologies.
Evolution of SRAM Cell Design From 6T to Advanced 4T Architecture in Memory ICs - Manufacturing Complexity and Cost Analysis of SRAM Cell Types
Examining the manufacturing process and associated costs of different SRAM cell types reveals a complex interplay between design complexity and financial implications. The traditional 6T SRAM cell, while proven in terms of its operational stability and performance, demands a larger silicon footprint and incurs a higher manufacturing cost due to its six-transistor structure. This complexity translates to a more intricate fabrication process and more steps required to assemble each cell. Conversely, 4T SRAM cells present a simplified design with just four transistors, leading to smaller cell sizes and potentially lower manufacturing hurdles. This streamlining of the design can theoretically translate to a reduction in fabrication costs. As the industry navigates smaller process nodes and higher integration densities, the need for streamlined fabrication processes becomes even more crucial. The shift toward designs like 4T highlights the ongoing challenge of finding that sweet spot between performance and manufacturing efficiency. It underscores the dynamic nature of SRAM design, where the evolution of cell architectures is intrinsically tied to balancing the demands of performance with the economic realities of production. The quest for better performance and higher density in memory technology invariably puts increased pressure on the manufacturing process to evolve and adapt, pushing designers to make tradeoffs in order to produce cost-effective yet efficient memory solutions.
The complexity of SRAM cells escalates when incorporating features to improve performance and reliability. For example, advanced 4T designs often rely on intricate feedback mechanisms to counter soft errors that become more common with smaller technology nodes. This intricacy adds to manufacturing costs and stretches out the design process.
While 4T SRAM cells are often touted for their area efficiency, their production involves meticulous material doping and strain management to uphold performance while shrinking cell sizes. This adds variability to production yields and costs.
The differences in read stability between 6T and 4T cells are often rooted in their structural differences. 4T designs necessitate careful tuning of current drive strengths to achieve competitive Static Noise Margins (SNM). This can make layout design more complicated and potentially decrease cost-effectiveness.
The constant scaling down of transistors presents unique difficulties for SRAM cell designs. Although 4T architectures adapt better to smaller nodes, the intricate interconnects often required to maintain performance can make manufacturing costs and processes more complex.
When we look at power consumption, while 4T cells often boast up to 30% lower static power compared to 6T, realizing these savings depends on finely tuning transistor parameters during manufacturing. This fine-tuning can drive up the initial costs.
Creating 4T SRAM cells, with their simpler read paths, might result in lower dynamic power consumption. But this often requires advanced lithography techniques and unique processing steps which can ultimately increase production expenses.
The shift towards 4T designs has emphasized the importance of Design-for-Manufacturability (DfM). This implicitly necessitates revamping existing manufacturing processes, which can require substantial time and financial resources as engineers adjust existing production systems for these new architectures.
It's important to note that the single-ended read scheme used in 4T SRAM cells represents a major departure from the conventional differential schemes in 6T designs. While it simplifies the circuitry, the transition requires thorough testing and validation, contributing to the overall manufacturing complexity and cost.
As memory densities climb, managing thermal stability becomes more challenging. 4T cells are engineered to dissipate heat efficiently, but this often requires unique materials or specialized processing during production, potentially increasing production costs and extending timelines.
Integrating advanced materials into 4T SRAM designs presents both opportunities and challenges regarding performance. While they can enhance speed and reduce energy consumption, their potentially higher costs and integration hurdles necessitate a thorough assessment of their return on investment in production settings. We must carefully consider if the benefits are worth the challenges.
Evolution of SRAM Cell Design From 6T to Advanced 4T Architecture in Memory ICs - Memory Access Speed and Read Write Operations Across Architectures
The speed at which memory can be accessed and the efficiency of read and write operations are key areas where SRAM architectures have seen notable changes. The shift from the established 6T SRAM design to the more recent 4T design illustrates a significant evolution in memory design principles. The 4T architecture has clear benefits, including a smaller footprint and lower energy consumption, due to its simplified structure and decreased capacitance.
While 4T can potentially enable faster access times and lessen certain types of read errors, it can also lead to some limitations in write speeds, particularly in scenarios that require high performance. Maintaining a balance between these aspects—performance, reliability, and efficiency—becomes increasingly important as the demand for higher memory density continues to grow. This evolving landscape necessitates the development of creative solutions that can push the limits of both speed and energy efficiency in the increasingly complex world of memory operations. As the industry continues to refine 4T designs, the ability to address these performance and efficiency trade-offs will remain a central focus in shaping the future of memory technologies.
SRAM cell access speed is surprisingly not solely determined by the number of transistors. In many situations, 4T cells can match or even surpass the speed of 6T cells due to their simpler structure. Reduced capacitance in 4T cells helps with faster switching, which is beneficial for performance.
Read and write operations in 4T SRAM designs seem less affected by issues related to read disturbances compared to 6T architectures. This enhanced reliability comes from their distinct read mechanisms, which are more resilient during high-frequency use.
Another interesting difference lies in power consumption. While 4T SRAMs generally consume less static power, they can fall behind 6T in dynamic performance, especially during demanding write operations. This is something to keep in mind when evaluating them for high-performance uses.
The change to advanced 4T designs has made us re-think the use of materials and doping in transistor construction, which has become especially crucial for them to remain functional with progressively smaller transistors. While beneficial for read stability and overall performance, this approach introduces complexity to manufacturing.
A notable practical advantage of 4T SRAMs is their enhanced thermal stability, enabling them to function at higher temperatures. This is a considerable benefit in high-performance computing where heat management is a major challenge.
The smaller size of 4T SRAM cells isn't just about increased memory density. It also opens the door for innovations like multi-level cell (MLC) structures, which could lead to higher-capacity memories in the future.
While better suited to scaling with smaller transistor sizes, 4T SRAMs still necessitate precise optimization of transistor dimensions and interactions. This is crucial for preventing performance drops as fabrication techniques improve over time.
The single-ended read operation in 4T SRAM simplifies circuit design and potentially offers faster read access compared to the differential read seen in 6T cells. This aspect might prove extremely helpful in environments demanding high performance.
The shift to 4T designs has also changed the way we think about Design-for-Manufacturability (DfM). Now, it's all about streamlined processes that accommodate modern production without compromising efficiency.
The balance between performance and power demands careful evaluation of the stability and efficiency of 4T SRAMs. While popular due to their smaller size, this search for the ideal balance is likely to result in even more innovative memory technologies in the future.
Revolutionize structural engineering with AI-powered analysis and design. Transform blueprints into intelligent solutions in minutes. (Get started for free)
More Posts from aistructuralreview.com: