Revolutionize structural engineering with AI-powered analysis and design. Transform blueprints into intelligent solutions in minutes. (Get started for free)
New Study Reveals Optimal Anchor Bolt Spacing for Multi-Story Buildings in Seismic Zones
New Study Reveals Optimal Anchor Bolt Spacing for Multi-Story Buildings in Seismic Zones - Optimal Anchor Bolt Spacing Range Identified for Seismic Zones
A new study has shed light on the optimal spacing for anchor bolts in multi-story buildings within seismic zones. While 3 meters has been a standard, this research suggests that a 4-meter spacing can offer comparable performance. The study's findings are particularly important given the crucial role of ductility in anchor bolt design for withstanding seismic forces. Furthermore, this research reaffirms the importance of sufficient embedment depth and staying abreast of evolving seismic design guidelines. These factors combined play a significant role in structural integrity during an earthquake. As engineers navigate the challenges of constructing in areas prone to seismic activity, these findings provide valuable insight for optimizing anchor systems in these critical structures.
A recent study on multi-story buildings in seismic zones suggests that there might be an ideal spacing for anchor bolts. The study, which delves into the impact of anchor bolt spacing on structural behavior during earthquakes, proposes an optimal spacing of 4 meters. This finding is intriguing, as it challenges conventional practices that often favor closer bolt spacing. The research highlights a potentially significant advantage of wider spacing, especially considering the minimal performance difference compared to a 3-meter spacing.
The study’s focus on anchor bolt spacing is based on the understanding that the performance of these critical elements during seismic events is crucial. It seems counterintuitive that increased spacing might improve a building's resilience during an earthquake, but this study suggests that the wider spacing could lead to more efficient load distribution and potentially enhance a building's ability to absorb and dissipate seismic energy. This finding encourages a reevaluation of established design philosophies for anchor bolts in high-risk areas. The optimal spacing, however, might not be a one-size-fits-all solution. The study indicates that factors like geographic location, soil conditions, and even the specific building materials used could play a significant role in determining the best anchor bolt spacing for a given structure.
While this research presents a compelling argument for adjusting conventional anchor bolt spacing practices, it’s essential to emphasize that further research and testing are crucial to fully understand the implications of this finding. The study's findings, combined with additional investigation, could lead to a shift in seismic design strategies and ultimately contribute to safer and more resilient structures in areas prone to earthquakes.
New Study Reveals Optimal Anchor Bolt Spacing for Multi-Story Buildings in Seismic Zones - Impact of Structural Systems on Lateral Load Performance
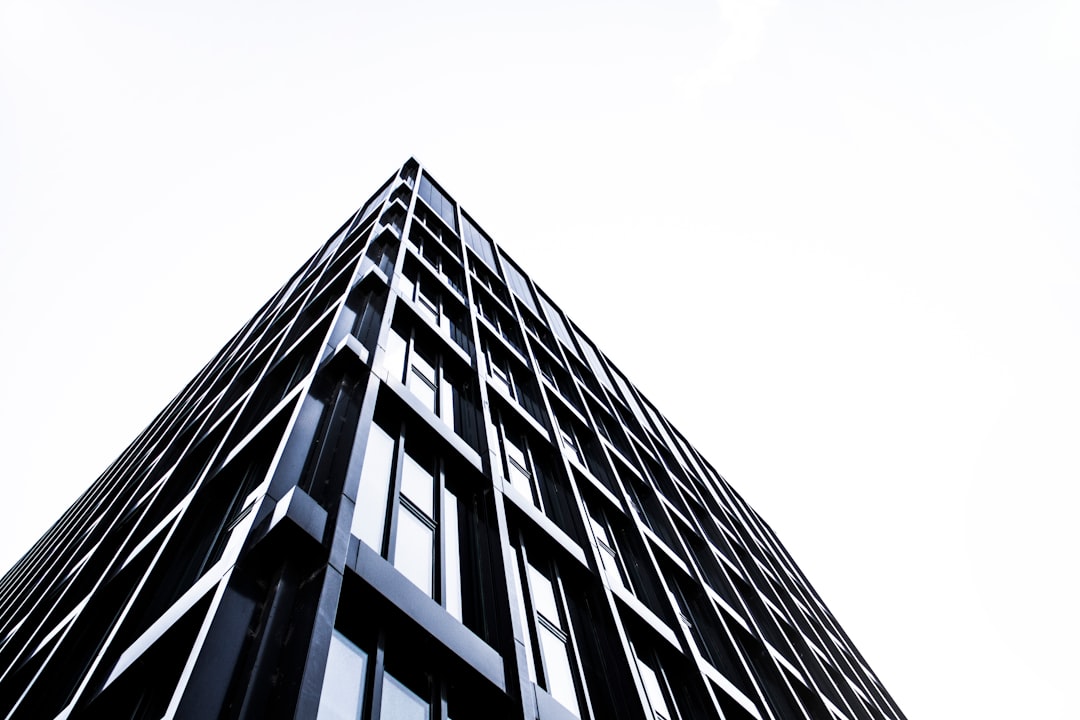
The way a building is structured has a huge impact on how it handles sideways forces, especially in earthquake-prone areas. Recent studies have been looking at different ways to build walls and frames to best resist these forces. One of the big things they've discovered is that special shear walls are extremely important for making buildings stronger during earthquakes. These walls help to absorb the energy from the shaking, making the building more likely to stay standing. There's also been a lot of work done using computer modeling to better understand how the different parts of a building interact during an earthquake. This allows engineers to design buildings that are more resistant to the specific challenges of seismic events.
A recent study exploring the relationship between anchor bolt spacing and seismic performance in multi-story buildings raises some interesting questions. While the study suggests that 4 meters might be a suitable spacing for anchor bolts in some cases, it’s crucial to consider the broader picture of structural behavior under lateral loads.
We must remember that ductility plays a significant role in mitigating seismic forces. While the study delves into anchor bolt spacing, understanding the influence of structural systems on a building's overall response to seismic events is equally important. Different framing systems, like moment-resisting frames, braced frames, and diagrid systems, respond to lateral loads in distinct ways. This variability impacts how seismic forces are distributed throughout the structure and subsequently, the effectiveness of anchor bolts. Furthermore, the study underscores the importance of considering vibration modes and first mode dominance. These factors, in conjunction with the impact of soil-structure interaction, can significantly influence the design and placement of anchor bolts.
Dynamic amplification, a phenomenon where structural elements experience amplified forces during an earthquake, must be accounted for. Anchor bolts need to be designed to effectively mitigate these forces. Moreover, the configuration of a building, its aspect ratio, and height-to-width ratio, are all crucial aspects influencing the design of the anchor bolt system. The materials used in construction, such as steel or concrete, also contribute to the building’s performance during a seismic event, impacting the effectiveness of anchor bolts.
The study's focus on anchor bolt spacing is a valuable starting point for understanding seismic design, but the importance of load path continuity cannot be overstated. Misalignment or discontinuities in anchor bolts can create points of stress concentration, potentially leading to structural failure. It’s vital to consider the cyclic loading effects of seismic events. The ability of anchor systems to withstand repeated loads without compromising their integrity is critical for ensuring structural stability. Examining the performance of structures in past earthquakes can shed light on the importance of research-backed design guidelines and highlight the consequences of inadequate anchor bolt spacing. This knowledge, combined with a deeper understanding of the interplay between structural systems, material properties, and vibration modes, is essential for creating safer and more resilient buildings in seismic zones.
New Study Reveals Optimal Anchor Bolt Spacing for Multi-Story Buildings in Seismic Zones - Thermal Performance Integration in Timber Building Seismic Design
Building with wood in earthquake zones presents a unique set of challenges. While timber is a sustainable and renewable material, it's essential to ensure its structural performance during seismic events. A new wave of research is focusing on integrating energy efficiency with seismic design in timber buildings, particularly light-frame structures. This integration aims to create buildings that are both environmentally friendly and resistant to earthquakes.
One promising approach involves using Cross Laminated Timber (CLT) panels, which can improve both seismic performance and energy efficiency. However, there's a delicate balance to strike. Increasing the thermal mass of a building, which is beneficial for cooling in hot climates, can actually negatively impact its seismic performance.
The complexity of analyzing the seismic behavior of timber structures can also be a barrier to wider adoption. Simplifying the design process while maintaining structural integrity and energy efficiency is crucial to making timber construction more viable in earthquake zones. This involves a thorough understanding of the interplay between thermal performance and seismic design principles, along with a commitment to developing building codes that reflect these complex relationships.
The study of anchor bolt spacing for multi-story buildings in seismic zones presents a fascinating starting point for understanding the complexity of seismic design. However, this research only scratches the surface of the interplay between a structure's thermal properties and its seismic resilience. Timber structures, with their unique thermal conductivity, present a compelling case study in how these two aspects interact.
One key aspect is the inherent insulating properties of timber, which can be beneficial in mitigating thermal bridging and potentially improving overall thermal performance in multi-story buildings. This, in turn, can affect how the structure reacts during seismic events. Another notable factor is timber's damping characteristics, which can contribute to reducing the amplitude of vibrations during earthquakes. This makes timber structures potentially more resilient to seismic forces.
In recent years, engineered wood products like cross-laminated timber (CLT) have emerged as promising reinforcements for timber structures. These materials offer a combination of thermal efficiency and structural strength, making them attractive for seismic zones. However, the thermal mass of timber itself can also influence the building's seismic response, especially in hot climates. Strategic placement of thermal mass components can impact temperature fluctuations and potentially affect building expansion and contraction during seismic events.
The evolving landscape of seismic design codes is starting to incorporate performance-based design methodologies specifically for timber structures. This is a significant shift that acknowledges the unique mechanical properties of timber and its behavior under seismic loads. It compels engineers to move beyond traditional design approaches.
The influence of temperature variations on structural performance is not limited to the global response of a building but also affects local behavior. Thermal expansions at connections can lead to differential movement and influence how loads are transferred during a seismic event. This requires careful attention to detail when designing connections in timber structures.
Advancements in numerical modeling offer more accurate simulations of the complex interactions between thermal and seismic forces in timber buildings. These models provide valuable insights for predicting how temperature variations can impact structural components during earthquakes. The integration of thermal and seismic considerations is also critical for anchor bolt design. Moisture content and temperature variations can influence the efficacy and placement of anchor bolts, necessitating a tailored approach in high-risk seismic zones.
Further complicating matters is the interaction between thermal and vibrational modes in timber structures. Understanding how thermal changes affect the natural frequencies of timber frames is crucial for accurate design under seismic loads. Dynamic response assessment, which considers the building's behavior under seismic loads, requires an understanding of its thermal behavior. Changes in temperature can influence stiffness and damping characteristics, potentially altering the anticipated seismic response.
Overall, there is a growing awareness of the importance of integrating thermal and seismic considerations in timber building design. This emerging research field offers promising avenues for achieving greater structural resilience in high-risk zones. However, more research and careful analysis are necessary to fully understand the intricate interplay between these two crucial aspects of building performance. Only then can we confidently design timber structures that effectively withstand both environmental and seismic challenges.
New Study Reveals Optimal Anchor Bolt Spacing for Multi-Story Buildings in Seismic Zones - Energy Dissipation Role of Damping Devices in High-Rise Structures
Damping devices play a crucial role in high-rise structures, particularly in seismic zones. They act as energy dissipaters, absorbing the shockwaves generated during an earthquake, which in turn reduces the impact on the building. This is achieved through the use of passive energy dissipation systems such as friction dampers, viscoelastic dampers, and hysteretic dampers. These systems have proven to be very effective, reducing the dynamic response of buildings by as much as 40-60% compared to traditional structures.
The optimal placement of damping devices is also a key consideration. The effectiveness of these systems tends to increase with the height and flexibility of the building, which is why they are particularly beneficial for tall, slender structures. This increased effectiveness allows for greater control over the building's vibrations during seismic events. The combination of damping devices and base isolation systems is also being investigated and may represent a major step forward in protecting high-rise buildings against seismic forces.
While much progress has been made in understanding the benefits of damping devices, further research is needed to continue improving their performance and integration into building designs. The focus on energy dissipation as a key design principle will be crucial to ensuring safer and more resilient high-rise structures in the future.
The role of damping devices in mitigating seismic forces in high-rise buildings is crucial, as these devices act as energy absorbers during an earthquake, significantly reducing the potential for structural damage. By converting kinetic energy into heat, damping devices help to minimize the peak lateral forces transmitted to the structure, which is crucial during an earthquake. Studies suggest that damping systems can effectively dissipate energy, leading to a significant reduction in the overall seismic response of high-rise buildings. The use of damping devices adds another layer of complexity to the design and modeling of high-rise structures, as engineers must carefully consider how these systems impact a building's dynamic response characteristics. One example is the use of Tuned Mass Dampers (TMDs), which are designed to counter building vibrations by oscillating out of phase with the structure's movement, potentially achieving a significant reduction in displacement during seismic events.
While damping devices are undoubtedly beneficial in improving the seismic resilience of high-rise buildings, there are trade-offs that engineers must consider. Factors such as increased weight, cost, and design complexity, require careful consideration. There is also a need for deeper understanding of how these devices impact the dynamic behavior of high-rise structures, influencing aspects like natural frequencies and mode shapes.
The integration of damping devices has allowed for a shift towards performance-based design in high-rise construction, enabling engineers to tailor a building's seismic resistance according to specific performance objectives rather than adhering solely to code minimums.
Some damping devices function as seismic isolation systems, effectively detaching the building from ground motion. This results in a significant reduction in the forces exerted on the superstructure and, as a result, improves occupant safety during an earthquake.
The selection of materials used for damping devices is critical for ensuring high energy dissipation properties without compromising durability. Ongoing research into novel materials and techniques holds the key to advancing the efficacy of damping devices.
While implementing damping systems can be an expensive proposition, a thorough cost-benefit analysis should be conducted to weigh the reduced risk of seismic damage against the initial investment and ongoing maintenance costs.
New Study Reveals Optimal Anchor Bolt Spacing for Multi-Story Buildings in Seismic Zones - Multiobjective Optimization Methods in Seismic Design
Multiobjective optimization methods are gaining traction in the seismic design of multi-story buildings. These methods excel at balancing competing goals like minimizing cost, maximizing earthquake resistance, and ensuring good structural performance. By using sophisticated tools like genetic algorithms and post-Pareto pruning, engineers can sort through a huge number of potential designs to find the very best ones. The growing power of computers has made these complex optimization methods much more practical, and they hold real promise for making buildings safer in areas prone to earthquakes. This new way of thinking about design highlights the need to consider all aspects of a building's performance to come up with solutions that work well in real-world situations.
Multiobjective optimization is becoming a significant tool in seismic design, as it allows for the simultaneous consideration of multiple competing goals. This approach is particularly valuable in seismic design because it recognizes the inherent trade-offs between different objectives, such as minimizing construction costs while simultaneously maximizing a building’s resilience to earthquakes. This duality, however, can be a significant challenge for engineers, who must navigate the complex interplay between different performance goals.
One of the key concepts in multiobjective optimization is the Pareto frontier. This represents the set of optimal solutions where no single objective can be improved without making another objective worse. By visualizing the Pareto frontier, engineers can understand the trade-offs involved in the design process and make informed decisions about balancing different performance priorities.
The range of optimization algorithms available for seismic design is extensive. Genetic algorithms and particle swarm optimization are just two examples, each with its own strengths and weaknesses. The effectiveness of any given algorithm can be highly dependent on the specific objectives being optimized, such as prioritizing weight reduction or controlling displacement. This variability highlights the importance of carefully selecting the appropriate algorithm based on the project's specific goals and constraints.
Sensitivity analysis plays a critical role in multiobjective optimization by helping engineers understand how changes in design parameters impact the overall performance of a building. Factors such as soil properties or loading conditions can significantly influence the design space. Therefore, conducting comprehensive sensitivity analyses is essential for assessing the robustness of a design under various conditions.
Adding stochastic elements, such as randomly varying ground motion or material properties, into the optimization process can make the resulting design more reliable. These probabilistic approaches help in addressing the inherent uncertainties associated with seismic evaluations. This is a crucial aspect of designing structures that can withstand the unpredictable forces of an earthquake.
Despite its advantages, multiobjective optimization presents significant computational demands, especially for complex structures. Engineers must carefully consider available computational resources and time constraints when selecting optimization methods. A balance needs to be struck between achieving the most comprehensive optimization and adhering to project deadlines.
An exciting development in multiobjective optimization is its integration with Building Information Modeling (BIM) frameworks. This integration allows for real-time feedback during the design process, enabling engineers to visually assess the impact of design changes on both performance and cost. This dynamic feedback loop can significantly enhance the efficiency and effectiveness of the design process.
The availability of historical earthquake data presents a valuable opportunity to improve the accuracy of predictive models used in multiobjective optimization. By incorporating data from past seismic events, engineers can better tailor their design choices, such as anchor bolt spacing, to specific seismic characteristics of a particular site.
Recent advancements in sensor technology open up the possibility of integrating real-time monitoring data into multiobjective optimization models. This could enable adaptive design strategies that respond dynamically to ongoing seismic activity, further enhancing a building's resilience.
Finally, the results of multiobjective optimization in seismic design could have a significant impact on regulatory standards and building codes. If widely adopted, these new strategies could lead to revisions in safety requirements, resulting in improved structural safety across various seismic zones. This is particularly important as our understanding of seismic design principles evolves.
New Study Reveals Optimal Anchor Bolt Spacing for Multi-Story Buildings in Seismic Zones - Topology Optimization Addressing Dynamic Load Uncertainties
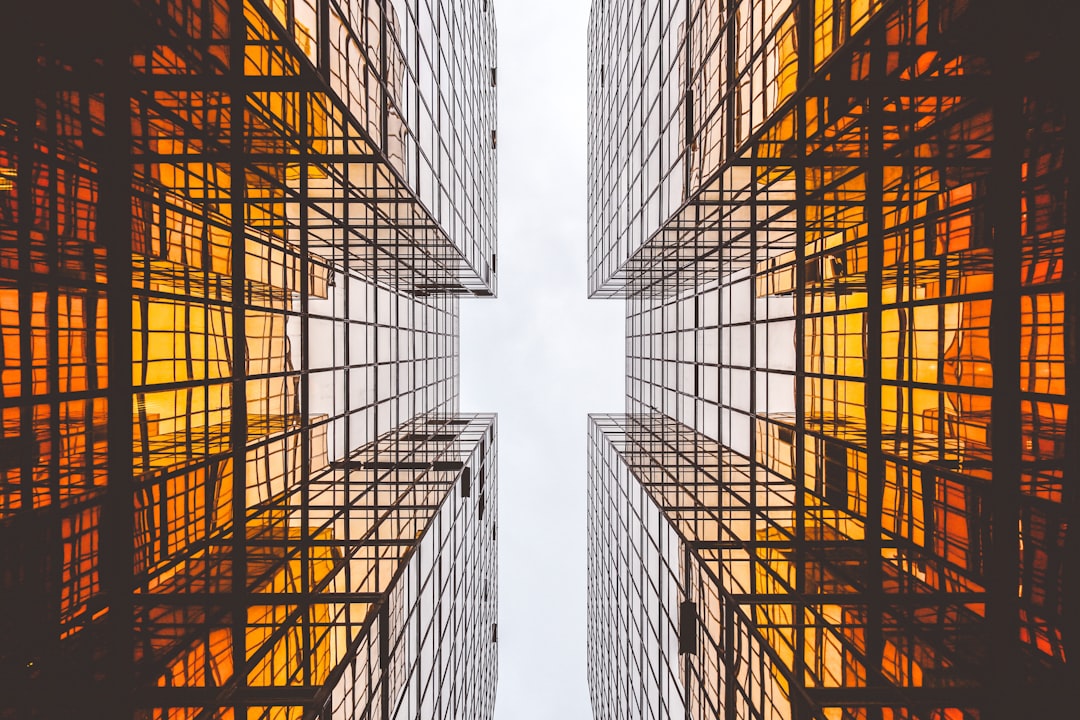
Topology optimization, a new approach to structural design, tackles the challenge of uncertain dynamic loads. This method focuses on finding the ideal shapes for structural components to withstand unpredictable forces, especially those generated by earthquakes. It takes into account variations in the strength and direction of these forces, ensuring buildings remain stable even under uncertain conditions. The use of robust optimization techniques further strengthens the design by minimizing not only average performance but also the potential variations in a structure's dynamic response, offering a higher level of safety and resilience against seismic events. This approach promises to make multi-story buildings significantly more reliable in earthquake-prone zones.
The study focusing on anchor bolt spacing provides a valuable starting point for understanding seismic design. However, the complex world of seismic forces requires a more nuanced approach, incorporating dynamic load considerations. Topology optimization, a powerful tool in structural engineering, has the potential to revolutionize how we design buildings to withstand earthquakes. This method goes beyond static analysis, allowing engineers to simulate real-world scenarios with varying dynamic loads. This approach addresses the nonlinear behavior of structures during earthquakes, which traditional methods often struggle to capture.
While computationally intensive, these techniques can generate designs that are not only efficient but also resilient. By incorporating uncertainty quantification, engineers can better assess the robustness of a design under unpredictable loads. This leads to a more accurate understanding of material distribution and the potential stress points within a structure. Topology optimization can also uncover possible failure modes before construction, allowing for proactive adjustments.
Furthermore, it's not just about finding the single "best" design; topology optimization often presents a range of feasible options. This opens up possibilities for more creative and efficient engineering solutions. The real power lies in the ability to design structures with adaptive characteristics, able to modify their response to real-time load changes.
The implications extend beyond seismic design. The principles behind topology optimization, coupled with dynamic load analysis, have applications in aerospace and automotive engineering, where performance under changing conditions is paramount. Ultimately, this approach represents a step towards more resilient and efficient structures in a world grappling with seismic hazards.
Revolutionize structural engineering with AI-powered analysis and design. Transform blueprints into intelligent solutions in minutes. (Get started for free)
More Posts from aistructuralreview.com: