Revolutionize structural engineering with AI-powered analysis and design. Transform blueprints into intelligent solutions in minutes. (Get started for free)
Structural Analysis of Philadelphia's 230M Gallon Daily Water Treatment Network Engineering Behind the Scenes at Baxter Plant
Structural Analysis of Philadelphia's 230M Gallon Daily Water Treatment Network Engineering Behind the Scenes at Baxter Plant - Daily Operations Processing 230M Gallons Through Three Main Treatment Plants
Philadelphia's water system, relying on three main treatment plants, daily handles a staggering 230 million gallons of water. This massive undertaking highlights the critical role these facilities play in the city's infrastructure. The ability of these structures to withstand the rigors of daily operations, while also adapting to pressures like climate change and growing regulatory hurdles, is paramount. The demand for consistent high-quality water output is rising as societal standards evolve, adding another layer of challenge. It's worth noting that the close link between energy use and water management has forced a new perspective on resource optimization. To stay ahead of these challenges, vigilant monitoring of water quality, ongoing training of plant operators, and creative solutions for managing the system's energy footprint are crucial. The success of this complex endeavor rests on the ability of the system to continually evolve and adapt to an ever-changing landscape.
Philadelphia's water system relies on three major treatment plants to process a massive 230 million gallons of water daily. This vast volume highlights the scale of the engineering challenge, especially when considering the intricate network of over 300 miles of pipelines required to distribute this treated water across the city.
One can't help but wonder how effectively the system handles the constant fluctuations in water quality. While the advanced monitoring systems allow for real-time adjustments, the inherent complexities of natural water sources remain a factor. Treatment plant operators must consistently adapt to changing contaminant levels, which can be influenced by weather patterns, industrial activity, and other external factors.
The reliance on chemical treatments like chlorine and alum, while effective, raises questions about their long-term impact on the water system and the environment. While coagulation and disinfection are essential, are there more sustainable alternatives currently being explored? The use of different technologies, such as membrane filtration and ozone treatment, across the plants suggests a commitment to finding optimal solutions, though whether this results in consistent water quality across the entire city is a concern that requires deeper investigation.
Furthermore, the energy footprint of such a massive operation is considerable. Energy recovery systems show promise for improving efficiency, yet it remains to be seen how significantly they will impact the overall energy consumption of these plants. Perhaps future developments in this area could significantly reduce the water system's ecological footprint.
The continuous testing and refining of the filtration process are a critical component of maintaining water quality. However, the development and implementation of new materials and methods must also be analyzed for their impact on overall operational efficiency. This research focus should not only be limited to cost reduction but also to a deeper understanding of potential drawbacks that might arise from utilizing new filtration materials.
Finally, compliance with EPA regulations, coupled with regular maintenance, are essential to ensure the system's reliability and public health. Yet, these aspects might present ongoing challenges. Addressing these challenges, and continuing to refine operational efficiency through methods like predictive maintenance, are crucial for the long-term success and sustainability of the system.
There are ongoing improvements and upgrades to the system. However, more detailed research and analysis are necessary for us to thoroughly understand and fully assess the current and future operational and environmental implications of the Philadelphia water system, especially in light of growing population and climate change related challenges.
Structural Analysis of Philadelphia's 230M Gallon Daily Water Treatment Network Engineering Behind the Scenes at Baxter Plant - Structural Design of Delaware River Intake System at Baxter Plant
The Delaware River Intake System at the Baxter Water Treatment Plant stands as a critical component of Philadelphia's water infrastructure. Its design is paramount, as it must withstand a variety of forces – from harsh wave action and strong currents to the pressures of ice buildup during winter. This robust design ensures the system's integrity during challenging weather conditions. The vast Delaware River watershed, extending across thousands of square miles, underscores the complexity of the site selection process and the necessity of meticulous structural analysis. The intake system not only needs to be structurally sound but also plays a significant role in maintaining the quality of the water that supplies the city. However, climate change introduces a new set of problems that need to be addressed with adjustments to both design and operations. The Baxter Plant's intake system demonstrates how the field of engineering must continuously adapt to changing environmental conditions while prioritizing both resilience and safeguarding water resources for the city.
The Baxter Plant's Delaware River intake system is a crucial component of Philadelphia's water supply, tasked with capturing water from the river and delivering it to the treatment facilities. It's fascinating how engineers have addressed the challenge of filtering out debris using bar racks and screens, which require regular maintenance to avoid operational issues caused by clogging. This aspect highlights a constant tension in the system – the need for continuous upkeep to ensure optimal performance.
The system's structural design relies heavily on reinforced concrete and steel, giving it the strength to handle the unpredictable forces of the river, including floods and variations in pressure. This choice is understandable, but one wonders if there might be alternative materials with improved sustainability or resilience that could be explored in future upgrades.
Hydraulic modeling plays a crucial role in the intake's design, allowing engineers to predict water flow and optimize the structure's placement. This minimizes disruptions to the natural river flow, which is essential to maintain a steady and reliable water supply. However, the complexities of the Delaware River's ecosystem make it essential to continue monitoring the impact of this structure on the broader environment.
A redundancy system built into the design adds another layer of complexity and reliability. If one component fails, others can step in to maintain water flow. This highlights a key consideration in critical infrastructure – how to mitigate the consequences of unexpected events. However, it does raise the question of how easily and efficiently such a complex redundancy system can be managed and maintained.
The intake's design includes strategies for managing sediment, with a settling basin designed to let larger particles settle before they enter the treatment processes. This approach protects downstream equipment, but we should consider how efficiently it handles variations in sediment loads, particularly during periods of heavy rainfall or river flooding.
The Delaware River's tides are a major factor in the intake's design. Engineers need to carefully calculate the system's depth to ensure it functions correctly at different tide levels. This emphasizes the importance of considering local environmental factors in designing infrastructure. However, are we truly considering the potential impacts of future sea-level rise on tidal influences in this region, particularly in light of climate change?
Real-time monitoring of water levels and flow rates using sensors allows for quick adjustments, improving efficiency and preventing overflows during peak flow periods. While this technology undoubtedly aids in optimization, the extent to which it truly optimizes the system's performance needs further investigation. Is there room for improvement in these monitoring and control systems to reduce the strain on the system and the operators?
The inclusion of variable frequency drives (VFDs) in the pump stations offers both energy efficiency and precise flow control based on demand. It's a smart design choice, but it raises the question of whether this technology is being utilized to its full potential and if any further improvements are possible within the system to enhance energy savings further.
Structural health monitoring (SHM) is implemented to detect and address potential issues proactively. It's a sensible approach, but it prompts further inquiry: how rigorous are these SHM protocols, and can they be refined for better prediction and mitigation of issues?
Finally, the foundation design is informed by extensive geotechnical investigations to ensure stability and prevent issues like erosion or subsidence over time. This approach highlights the necessity of deep understanding of local soil conditions when designing structures for long-term performance. However, with a changing climate, we must ensure that the long-term stability of the foundation design accounts for potential shifts in soil conditions.
In summary, the Delaware River intake system at the Baxter Plant is a complex and sophisticated piece of engineering, addressing several critical aspects of water supply and treatment. However, we should continue questioning the effectiveness of current design choices and the long-term sustainability of this system. This critical perspective will help ensure that the Baxter Plant, and subsequently Philadelphia’s water system, remains resilient and reliable in the face of future challenges.
Structural Analysis of Philadelphia's 230M Gallon Daily Water Treatment Network Engineering Behind the Scenes at Baxter Plant - $125M Basin Construction Project Expanding Treatment Capacity
Philadelphia's water treatment network is undergoing a major upgrade with the $125 million Basin Construction Project at the Baxter Water Treatment Plant. This project focuses on increasing the plant's capacity to process the city's water supply. A new, one-acre basin, built 40 feet underground, will serve as a temporary storage area before water enters the treatment process. This expansion is part of a larger, $5 billion, 25-year Water Revitalization Plan that includes about 400 projects to improve and expand the city's water infrastructure. The plan also includes updated disinfection methods to meet current EPA standards. This project, though significant, is only a piece of the puzzle in addressing the growing need for high-quality water amidst a changing climate. The long-term impact of climate change and the ever-increasing demands placed on the system require ongoing evaluation and adjustments to ensure the sustainability of Philadelphia's water supply.
The $125 million Basin Construction Project at the Samuel S. Baxter Water Treatment Plant represents a significant investment in Philadelphia's water infrastructure. It's part of a larger, 25-year, $5 billion Water Revitalization Plan encompassing around 400 projects aimed at improving and expanding the city's water treatment facilities. While this particular project focuses on increasing treatment capacity by roughly 20% – adding around 50 million gallons to the Baxter Plant's existing 230 million gallon daily output – it raises questions about the long-term scalability of the current treatment methods.
This new basin, spanning an acre and extending 40 feet below grade, acts as a holding tank for raw water before it undergoes treatment. The project integrates modern monitoring technologies to allow for real-time assessments of water quality. However, the effectiveness of these systems needs to be carefully scrutinized, especially across different seasons and water conditions, to ensure they accurately reflect treatment performance. The chosen engineering materials for the project emphasize longevity. But their long-term durability when facing the harsh chemicals used in the water treatment process and their fatigue resistance over decades need further examination.
The basin's complex design incorporates intricate hydraulic models to manage water flow. These models need continuous calibration to adapt to fluctuations in water quality and quantity, ensuring the engineered solutions remain effective. The project includes backup systems, or redundancies, designed to safeguard against potential failures. Yet, the practical impact of these redundancies on system reliability and the associated maintenance needs require careful evaluation.
The design also accounts for increased sediment loads that might stem from the upgrades. But it remains uncertain if the current sediment management techniques are sufficient to handle future challenges. Variable frequency drives (VFDs) are incorporated into the new construction to optimize pump operation and improve efficiency. However, there's a need to explore how effectively the system can optimize these VFDs for the actual, fluctuating water demands.
The foundation of the new basin is based on extensive geotechnical studies to address the potential variability of soil conditions. This underscores the need for accurate predictive models for long-term ground stability. While the project addresses the current need for increased capacity, its design also needs to accommodate future expansion and growth beyond the current projections. How well the design allows for future adaptation should be a critical factor throughout the project's lifecycle.
In conclusion, the Basin Construction Project is a significant step in the modernization of Philadelphia's water infrastructure, but continued research and analysis are crucial. Understanding the project's long-term implications for operational efficiency, environmental impact, and adaptability to future demands will be essential for ensuring the long-term success and sustainability of the Baxter Plant and the entire Philadelphia water system.
Structural Analysis of Philadelphia's 230M Gallon Daily Water Treatment Network Engineering Behind the Scenes at Baxter Plant - 2024 Status of Torresdale Station 70 Year Infrastructure Update
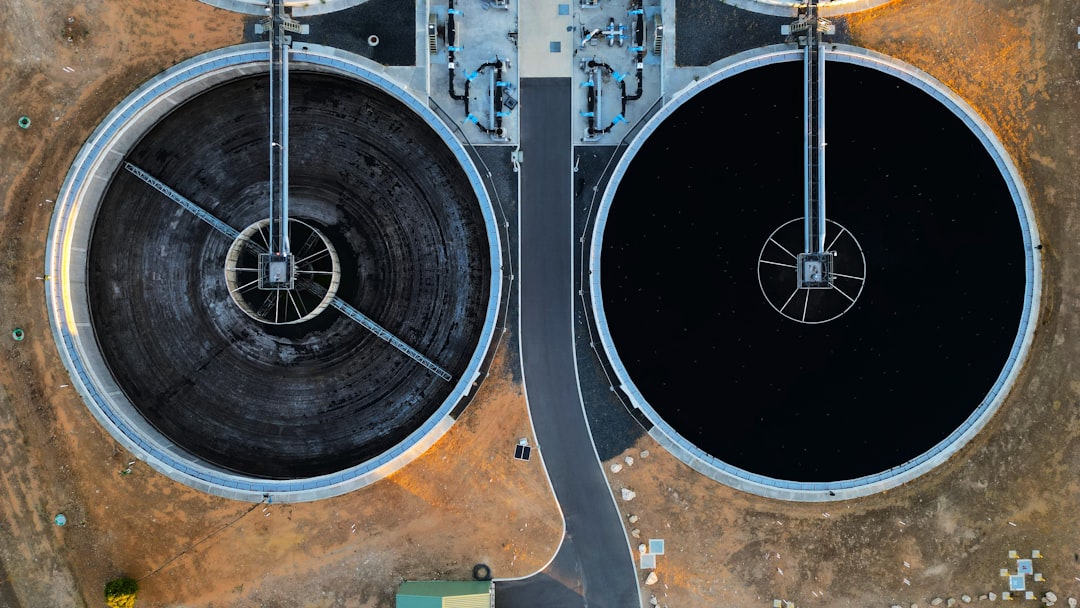
The 2024 status of the Torresdale Station's 70-year infrastructure update signifies a crucial point in Philadelphia's ongoing water infrastructure renewal project. Torresdale, a pivotal part of the city's water treatment network, is currently undergoing a thorough structural analysis as part of the comprehensive 25-year Water Revitalization Plan. This plan tackles the modernization of water systems, many of which were initially built over 70 years ago. The initiative is backed by a significant financial investment, including a substantial low-interest EPA loan of $340 million, highlighting the pressing need to address aging facilities that are responsible for handling Philadelphia's massive daily water supply of 230 million gallons. While progress is being made, concerns linger about the effectiveness of these improvements in the face of evolving environmental challenges like climate change and increasingly stringent regulatory requirements. It's vital that the ongoing assessment and modernization efforts at Torresdale prioritize building not just systems that address current demands, but also those that can withstand future challenges and ensure long-term water security for the city.
Torresdale Station, a vital part of Philadelphia's water treatment network, has seen a considerable transformation since its construction 70 years ago. The station has transitioned from its initial, simpler filtration methods to a more complex multi-stage treatment process, reflecting the increasing demands for high-quality drinking water. The 2024 infrastructure upgrade at Torresdale aimed to further refine its capabilities, expanding its daily output by an additional 30 million gallons. This expansion gives Torresdale a slightly larger role in the city's overall water treatment capacity.
One interesting aspect of the recent updates is the use of a new composite material for the basin linings. This material offers superior resistance to chemical corrosion compared to what was used in the past, promising a longer lifespan for the basins, especially given the exposure to the harsh chemicals involved in water treatment. The station's monitoring capabilities have also been significantly enhanced. The new real-time water quality monitoring systems incorporate predictive analytics, allowing operators to potentially foresee contamination events based on past data and operational conditions. This predictive element is a significant step forward compared to the reactive approaches of the past.
Furthermore, the 2024 upgrades addressed seismic considerations that were less prominent in the initial design. Adhering to contemporary seismic standards, the plant now features improvements to enhance its resilience in the event of an earthquake. It's interesting to note that seismic design is increasingly important, likely due to a heightened awareness of seismic activity in the region, or perhaps it reflects broader national building code changes. Energy efficiency has also become a key focus. Torresdale now integrates energy recovery techniques that leverage the kinetic energy of water flow. While it remains to be seen how impactful these energy recovery mechanisms are in the long run, they certainly suggest a growing awareness of the need for resource-efficient operations in the water treatment sector.
Redundancy is another theme in the latest updates. The new design incorporates dual-redundancy for key components, offering a backup system in the event of a primary system failure. This redundancy is becoming a common practice in critical infrastructure, but it does raise questions about the complexity of managing and maintaining such a system, particularly when the equipment ages. Sediment management has also received attention. Updated sedimentation basins utilize advanced mechanical agitation, aiming for improved efficiency in removing sediment. However, it's uncertain if this will be truly effective in handling a wide range of sediment loads, especially during periods of intense rainfall or river flooding.
The Torresdale upgrade also included incorporating a sophisticated geotechnical monitoring system. This system provides continuous soil stability information, a sensible precaution in light of recent extreme weather events that might impact soil integrity.
In the larger context of the Philadelphia water network, the Torresdale Station upgrade underscores the challenge of balancing the integration of modern technologies with the reality of a gradually aging infrastructure. While the upgrades are positive, it highlights the pressing need for ongoing assessment and analysis of the system. The long-term viability and efficiency of these systems, along with the integration of new technologies in older parts of the network, are key challenges to ensure the water system remains functional for years to come.
Structural Analysis of Philadelphia's 230M Gallon Daily Water Treatment Network Engineering Behind the Scenes at Baxter Plant - Technical Analysis of Multi Stage Filtration and Chemical Treatment Process
The technical analysis of multi-stage filtration (MSF) and chemical treatment processes within a water treatment network reveals both promise and potential drawbacks. This approach, particularly useful for smaller communities and those seeking greater efficiency, surpasses conventional methods in achieving water quality goals. Performance indicators, such as flow rates and performance ratios, show the potential for improvements in overall system efficiency. Further, MSF processes contribute to significant cost reductions, potentially lowering water prices considerably. This benefit aligns with global efforts to provide safe drinking water, particularly in underserved areas. The technology is well-suited for rural and smaller water treatment plants that face financial and resource constraints.
However, the increasing utilization of various chemical treatments during these processes raises environmental concerns. The growing reliance on an array of chemicals requires a thorough evaluation of the long-term consequences. This includes the evaluation of chemicals currently in use, but also the potential impact of newer treatment methods and materials on both the water and the environment. It's critical to address how well these methods, while promising, can actually adapt to evolving water quality challenges and ensure the water remains safe for public consumption. While operational efficiency can improve with these new approaches, it is important to assess the sustainability of these processes for the long-term health of both the environment and communities relying on the water supply.
Multi-stage filtration, a cornerstone of Philadelphia's water treatment strategy, is essential for achieving the stringent safety standards set by the EPA. Unlike traditional methods, which might rely on a single approach, this layered system employs a blend of physical, chemical, and biological processes to tackle a wider range of contaminants effectively. It's worth questioning whether this comprehensive approach is truly necessary or if it's potentially overengineered for the specific characteristics of the Delaware River water.
At the Baxter Plant, chemical treatment relies heavily on advanced techniques like coagulation and sedimentation. Aluminum sulfate (alum) plays a crucial role in destabilizing and clumping particles, making their removal easier. Research suggests that fine-tuning the alum dosage and mixing intensity significantly impacts the effectiveness of these steps, ultimately affecting the water's overall quality. This calls for ongoing refinement of these processes, possibly leading to more sustainable and efficient solutions.
Membrane filtration, a vital part of the multi-stage approach, utilizes porous membranes to filter out incredibly tiny particles, down to 0.1 micrometers. This capability is crucial for dealing with emerging contaminants like pharmaceuticals and personal care products, often resistant to standard treatment methods. While the technology seems promising, questions arise about the potential accumulation of these filtered contaminants and the long-term disposal strategy for these membrane filters.
Real-time monitoring, integrated with advanced sensors and data analytics, provides constant feedback on water quality and treatment effectiveness. This allows operators to make swift adjustments to chemical dosages and filtration rates, improving efficiency and potentially reducing chemical waste. However, it's unclear how robust these monitoring systems are and whether they can effectively handle unforeseen events or extreme variations in water conditions.
The hydraulic design of sedimentation basins is vital for efficiently separating solids from liquids. Fluctuations in water flow rates can severely affect sedimentation performance, demanding meticulous calibration of hydraulic parameters. Understanding the complexities of these hydraulic designs and how they influence the overall treatment performance is a crucial area of future investigation.
Redundancy is a cornerstone of the Baxter Plant's chemical treatment systems, with backup components in place for critical parts like pumps and mixers. This strategy ensures uninterrupted operation, highlighting a strong commitment to resilience in water treatment infrastructure. Yet, it begs the question of how easily manageable and maintainable this complex redundancy strategy is over the long term.
The possibility of using alternative coagulants, such as polyaluminum chloride (PAC), is increasingly attracting interest due to its improved performance at lower temperatures and reduced chemical residue in treated water. This might improve downstream treatment processes. It's important to continue investigating the environmental implications of transitioning to new coagulants and ensure that the potential benefits outweigh any unforeseen risks.
Activated carbon filters are often included in the filtration process due to their strong adsorption properties, especially in removing dissolved organic contaminants. However, these filters need regular replacement, which raises concerns about the lifecycle management of the filter material and its environmental impact. A deeper understanding of the long-term implications of using these materials is vital for sustainable water treatment.
Ozonation, a recent development in chemical treatment strategies, not only disinfects but also minimizes the formation of disinfection byproducts (DBPs). While it's effective at breaking down harmful organic compounds, controlling the ozone dosage is critical to avoid any unintended side effects during treatment. We must weigh the benefits of ozonation against the potential risks associated with ozone's use.
Research indicates that interactions between treatment stages can lead to unforeseen consequences, like the release of contaminants previously trapped during the filtration process. This emphasizes the need for continued research to fine-tune each stage of the treatment process. Finding ways to optimize each step can improve overall system performance while mitigating the chance of these unforeseen issues.
Ultimately, Philadelphia's multi-stage water treatment system is a sophisticated endeavor designed to protect public health. However, the field is constantly evolving, and it's important to critically examine its efficiency, effectiveness, and long-term sustainability. A continuous cycle of questioning, research, and refinement is essential to ensure that the water treatment process remains optimized for the city's needs today and for future generations.
Structural Analysis of Philadelphia's 230M Gallon Daily Water Treatment Network Engineering Behind the Scenes at Baxter Plant - Climate Adaptation Engineering Solutions for Philadelphia Water Network
Philadelphia's water network, serving over 1.6 million residents, faces increasing pressure from climate change. To address this, the Philadelphia Water Department (PWD) launched the Climate Change Adaptation Program (CCAP) in 2014. The CCAP's primary aim is to minimize the risks and expenses associated with climate change impacts on the city's water systems, including drinking water, wastewater, and stormwater management. This involves sophisticated analyses of potential climate scenarios and how they might affect existing water infrastructure.
PWD's strategy for climate adaptation relies on a multi-pronged approach. It embraces both conventional "gray" engineering solutions, like infrastructure upgrades, and more nature-based, or "green," solutions. This combined approach allows for flexible and context-specific responses to climate-related challenges, which can range from altered precipitation patterns to increased flooding risks. The PWD's focus on integrating climate change considerations into its planning processes is noteworthy. It underscores a growing awareness of the vital role that effective water infrastructure plays in a city's ability to adapt to an increasingly volatile climate.
However, the complexities of climate change are constantly evolving. PWD's approach, while promising, needs ongoing scrutiny to guarantee the continued effectiveness and efficiency of the city's water infrastructure. This is critical to ensuring that the water supply remains reliable and safe for the city's residents. PWD's commitment to resilience and sustainable water management practices positions it as a potential leader in the ongoing efforts of American water utilities to adapt to a changing climate.
Philadelphia's water infrastructure, with components dating back 70 years or more, especially at Torresdale Station, is facing the challenge of adapting to a changing environment. These aging systems require consistent updates to mitigate the effects of time and water quality fluctuations that can lead to structural degradation over time.
The new basin at Baxter highlights a move towards advanced materials. These materials, like the new composite liners, are engineered to resist corrosion from the intense chemical treatments and wear and tear caused by the high sediment loads during operation, offering a potential increase in longevity compared to the materials used in older water treatment facilities.
The Baxter Plant has adopted a more proactive approach to managing water quality through advanced monitoring. Predictive analytics combined with real-time data provide plant operators the ability to make adjustments to proactively mitigate potential issues before they cause larger problems. This represents a shift from relying solely on reactive measures to actively prevent contamination.
The installation of Variable Frequency Drives (VFDs) in Baxter's pump stations demonstrates a sophisticated approach to energy efficiency. These systems allow pumps to operate at optimal levels depending on water demands, ensuring the most efficient use of energy while simultaneously allowing the system to react quickly to fluctuating flow conditions.
Climate change requires a shift in how we design for the future. Philadelphia's water management plans are now integrating hydraulic modeling that helps plan for future scalability of the water treatment processes, going beyond simply meeting present demands. The aim is to ensure the system can evolve as the city's needs and the effects of climate change evolve over time.
The use of incremental agitation techniques within the sedimentation basins at Baxter reflects an attempt to enhance the system's resilience to variable water conditions. This approach is especially relevant during intense rainfall, when increased sediment loads can strain the system's capacity to remove impurities.
Building codes and structural design principles have evolved. Seismic upgrades at Torresdale reflect a growing awareness of earthquake risks in the region, or perhaps it's due to broader national design standards. These upgrades increase the system's ability to withstand unexpected events.
The adoption of structural health monitoring (SHM) systems shows an evolution in how the city approaches the upkeep of its critical infrastructure. These systems continually monitor the integrity of the structures, giving operators the ability to address potential issues before they escalate into costly repairs or service disruptions. This strategy promotes proactive maintenance over reactive repairs.
Redundancy plays an increasingly important role in modern critical infrastructure planning. The Philadelphia water system includes redundancy in various parts of its network. These backups provide resilience against unforeseen failures, ensuring that even if one component fails, the system can continue to operate effectively. However, this design feature also adds another layer of complexity to the management and maintenance of the system.
Ongoing research into chemical and physical treatment processes is critical. Instead of simply fixing current issues, Philadelphia's water network is designed to be adaptable, continuously improving how it addresses present and future challenges. This includes the continual evaluation of treatment methodologies and material choices for a sustainable approach to providing water in a changing climate. The need to constantly assess the long-term effectiveness of these methods remains critical as demands and conditions continue to evolve.
Revolutionize structural engineering with AI-powered analysis and design. Transform blueprints into intelligent solutions in minutes. (Get started for free)
More Posts from aistructuralreview.com: