Structural Integrity Risks How Dissolved Solids in Water Accelerate Steel Reinforcement Corrosion in Concrete Foundations
Structural Integrity Risks How Dissolved Solids in Water Accelerate Steel Reinforcement Corrosion in Concrete Foundations - Electrochemical Testing Reveals 30% Faster Steel Corrosion in High TDS Environments
Electrochemical studies have shown that corrosion in steel can progress up to 30% faster when exposed to environments with high concentrations of dissolved solids. This accelerated corrosion is particularly noticeable when chloride ions from dissolved salts are present, leading to concentrated forms of corrosion like pitting and crevice corrosion. The consequences of this accelerated corrosion are significant for structures built with reinforced concrete, such as foundations. These structures can experience premature degradation, requiring costly repairs and potentially compromising structural integrity.
Given the growing economic burden associated with corrosion in concrete, these findings highlight the need for improved methods to assess corrosion and a more detailed understanding of how dissolved solids impact corrosion processes. Effectively mitigating the effects of high TDS environments is essential for ensuring the durability and safety of concrete structures over their intended lifespan.
Recent research using electrochemical methods has shown that steel corrosion in environments with high total dissolved solids (TDS) can proceed up to 30% faster than in typical settings. This acceleration is primarily attributed to the increased concentration of dissolved salts, especially chloride ions, which are known to disrupt the protective oxide layer that normally forms on steel surfaces. This disruption makes the steel more vulnerable to corrosion, potentially leading to faster deterioration and a shorter lifespan for reinforced concrete structures.
The implications of this accelerated corrosion are substantial. The increased corrosion rate can lead to unexpected and costly maintenance issues and even structural failure over time. Furthermore, high TDS waters can create conditions that encourage galvanic corrosion, where different metals within a structure form localized electrochemical cells, leading to rapid deterioration. It's been observed in laboratory studies that corrosion pits in high TDS environments are often considerably deeper, sometimes up to 50% deeper, than those formed in lower TDS settings, highlighting the heightened risk to structural integrity.
It's also important to note that the relationship between TDS concentration and corrosion rate is not linear. Even relatively small increases in dissolved solids can have significant, if not dramatic, effects on corrosion over time. This underscores the need for a careful consideration of water chemistry when dealing with concrete structures. Other ions besides chlorides, such as sulfates and nitrates, can also play a role in accelerating the corrosion process. They contribute through chemical reactions that degrade the protective film on the steel surface. Temperature also acts as a significant factor, as warmer water can make high TDS environments more aggressive, further escalating corrosion rates.
The disruption of the passive film – a protective barrier – on the steel surface is central to this accelerated corrosion in high TDS settings, which, as a result, makes pitting and uniform corrosion more prevalent. Understanding these complex interactions is crucial to improving the durability of reinforced concrete. Engineers and designers must recognize the significant impact of TDS when formulating concrete mixes and selecting materials for specific environments, especially those with variable water quality. Failing to do so may result in premature degradation and unexpected failures of our infrastructure.
Structural Integrity Risks How Dissolved Solids in Water Accelerate Steel Reinforcement Corrosion in Concrete Foundations - Water Penetration Through Micro Cracks Doubles Concrete Foundation Deterioration Rate
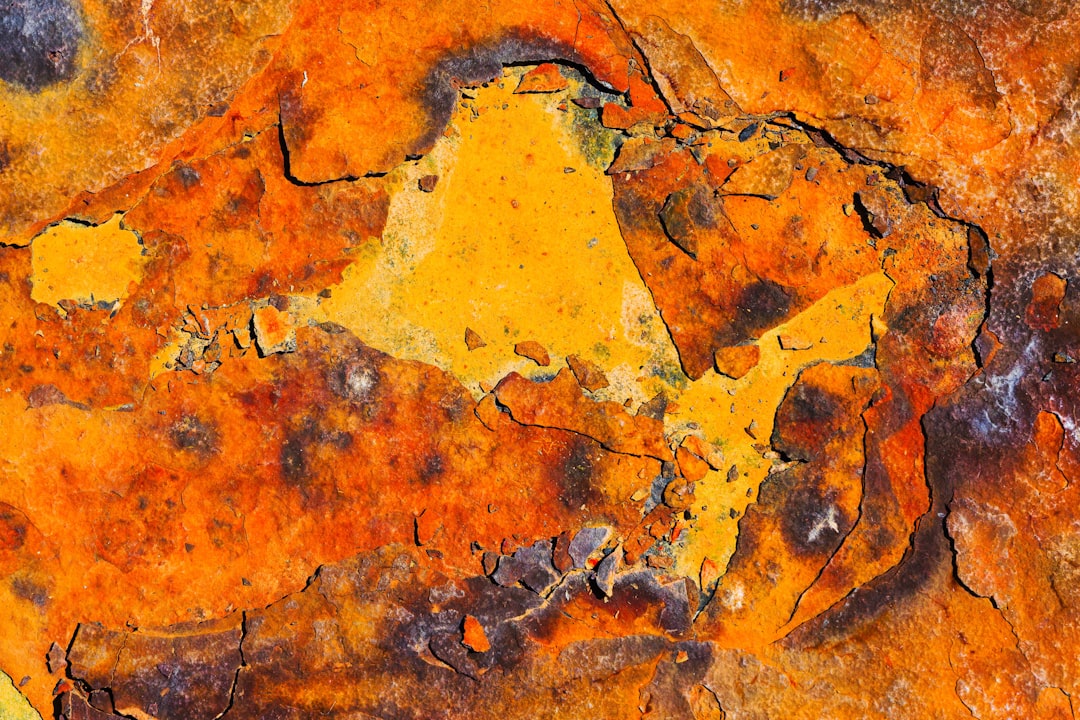
The presence of tiny cracks, or microcracks, within concrete significantly impacts its durability. These microcracks can effectively double the rate at which concrete deteriorates by providing pathways for water to penetrate the material more easily. This increased water penetration not only weakens the concrete's ability to keep water out, but also allows harmful substances like chloride ions to enter and reach the embedded steel reinforcement. The consequence of this is a heightened risk of corrosion of the steel, which can severely compromise the structural integrity of the foundation.
It's been observed that the ease with which water can move through concrete, or its permeability, can increase dramatically. This is particularly true when the initial mix of water and cement varies even slightly. Increased permeability makes it even easier for water to enter through these microcracks, leading to further deterioration. It is crucial to understand the relationship between the microcracks, water infiltration, and the resulting corrosion in order to maintain the structural integrity of concrete foundations over time. By understanding these processes, engineers and designers can develop building methods that increase the resilience of concrete structures and minimize the risk of rapid degradation.
The presence of microscopic cracks in concrete, often less than a tenth of a millimeter wide, can dramatically accelerate the deterioration process, compounding the already concerning issue of steel corrosion caused by dissolved solids in water. This accelerated deterioration arises from the increased permeability of the concrete due to these micro-cracks, effectively creating pathways for water and the dissolved salts it carries to reach and interact with the embedded steel reinforcement. It appears that water ingress can be significantly increased through these tiny fractures, leading to a doubling, or even greater, increase in the rate of concrete deterioration.
These micro-cracks are not just a nuisance, they can significantly compromise the integrity of foundations and other concrete structures. They act as conduits for corrosive agents such as chloride and sulfate ions, allowing them to penetrate the concrete far more readily than through intact material. This increased access to the steel reinforcement greatly accelerates the corrosion process, contributing to the premature degradation of the structural elements. While we previously focused on the impact of dissolved solids themselves, the role of water transport through micro-cracks adds another layer of complexity to the corrosion process.
The presence of these cracks not only allows for rapid water ingress, but it can also create observable signs of deterioration on the concrete surface. Staining and efflorescence, a white powdery deposit, are visual indicators that can suggest the presence of these micro-cracks and potentially underlying structural problems. Sadly, these surface manifestations can sometimes be ignored until more severe deterioration has occurred.
Although water is crucial for the initial curing of concrete, its sustained presence over time can become highly problematic. Acting as a transport medium for harmful ions, it facilitates corrosion and greatly intensifies the damage from high Total Dissolved Solids (TDS) environments. Recent studies suggest that water flowing through these micro-cracks can transport dissolved solids into the concrete at rates exceeding initial estimations. These dynamic interactions are challenging to predict, which highlights the need for adaptive, rather than static, maintenance approaches for infrastructure.
The rate at which these micro-cracks contribute to deterioration is a complex function of crack size, distribution, and environmental factors such as temperature and humidity. These factors can influence water penetration rates, potentially causing more rapid corrosion than anticipated. Interestingly, the formation of these cracks can arise from a variety of stresses, including temperature changes, concrete shrinkage, and structural loads, further complicating our understanding of this phenomenon. These stress-induced micro-cracks might be initially undetectable, only becoming visible when significant damage has already occurred.
Current repair strategies need to evolve to more effectively address the issue of micro-cracks and water ingress. Rather than merely patching visible cracks, a more holistic approach is needed, utilizing advanced materials and techniques to create hydrophobic barriers. Such barriers would prevent water penetration and the subsequent transport of corrosive agents.
It’s quite evident that the interplay between micro-cracks and high TDS environments is intricate and non-linear. For example, even a small influx of salts from de-icing agents, normally not a cause for concern, can lead to substantial and rapid corrosion growth when facilitated by water penetration through micro-cracks. The challenge for us is to better understand and model these intricate interactions.
As we refine our understanding of the influence of micro-cracks on structural performance, engineers need to adapt traditional design practices and incorporate insights from recent studies into future projects. This incorporation is crucial to developing predictive models for long-term deterioration, allowing us to design and build more resilient infrastructure that can withstand the challenges of exposure to high TDS water and the inevitable occurrence of micro-cracking. Ultimately, it's the integration of these insights that will lead to the improvement of long-term structural integrity.
Structural Integrity Risks How Dissolved Solids in Water Accelerate Steel Reinforcement Corrosion in Concrete Foundations - Ion Migration Patterns Show Critical pH Changes Near Steel Interface
Investigations into how ions move near the steel surface within concrete structures have revealed important changes in pH levels. These pH fluctuations are especially significant because they can strongly affect the structural integrity of concrete. When chloride ions, often found in environments with high concentrations of dissolved solids, reach the interface between the steel and the concrete, they can disrupt the natural protective layer on the steel, accelerating corrosion. This change in pH not only supports the formation of substances that contribute to corrosion, but it also increases the chances of premature structural failures in concrete foundations. Gaining a deeper comprehension of how these ions behave is critical for developing techniques that can improve the longevity and structural soundness of reinforced concrete. The intricate nature of the boundary between the steel and the concrete highlights the need for more sophisticated techniques to analyze these interfaces and minimize the risk of corrosion. This is a key part of protecting the long-term strength of our built environments.
Ion movement patterns close to the steel reinforcement in concrete can result in significant shifts in pH, a critical factor influencing corrosion. These pH variations impact the stability of corrosion products, which in turn affect the pace of steel corrosion within the concrete structure. Notably, as pH decreases near the steel, the likelihood of localized corrosion, such as pitting, increases, leading to potentially rapid and severe degradation.
The cyclical fluctuations in pH, caused by ion migration, are also concerning, as they can lead to unstable protective layers on the steel surface. These fluctuations can contribute to periods of heightened reactivity and corrosion. The formation of localized electrochemical cells at the steel-concrete interface can be further impacted by specific ions in the water and surrounding environment, leading to variations in corrosion across the steel surface.
Furthermore, ion migration can elevate the concentration of hydrogen ions near the steel, potentially resulting in a phenomenon called hydrogen embrittlement. This can severely weaken the steel without obvious external signs, increasing the risk of sudden, unexpected structural failures.
The depth that these ions penetrate into the concrete significantly impacts the performance of any protective barriers that are used. Unexpectedly, factors such as concrete density and moisture levels can greatly influence the depth to which corrosive substances penetrate, further highlighting the importance of thorough testing during the design phase of any structure.
Moreover, temperature plays a significant role in the speed at which ions move. Higher temperatures can accelerate ion migration and corrosion rates, requiring engineers to carefully consider material choice in various environments. The roughness of the steel surface itself also affects the impact of pH changes and ion movement by creating microscale environments that trap moisture and corrosive ions, leading to increased localized corrosion.
However, directly monitoring and measuring ion movement and pH changes at the steel-concrete interface is exceptionally challenging. Conventional, non-destructive testing methods may not fully capture the complexity of these processes, necessitating ongoing monitoring strategies. Finally, the specific type of aggregates in the concrete can change the rates at which ions migrate and alter the surrounding pH. It is clear that engineers must take great care in selecting aggregates for use in environments with high total dissolved solids to minimize potential corrosion risks. Understanding these complex interactions is critical for accurately predicting long-term structural performance and ensuring the integrity of reinforced concrete structures in diverse environments.
Structural Integrity Risks How Dissolved Solids in Water Accelerate Steel Reinforcement Corrosion in Concrete Foundations - Temperature Impact Studies Link 15°C Rise to 40% Corrosion Acceleration
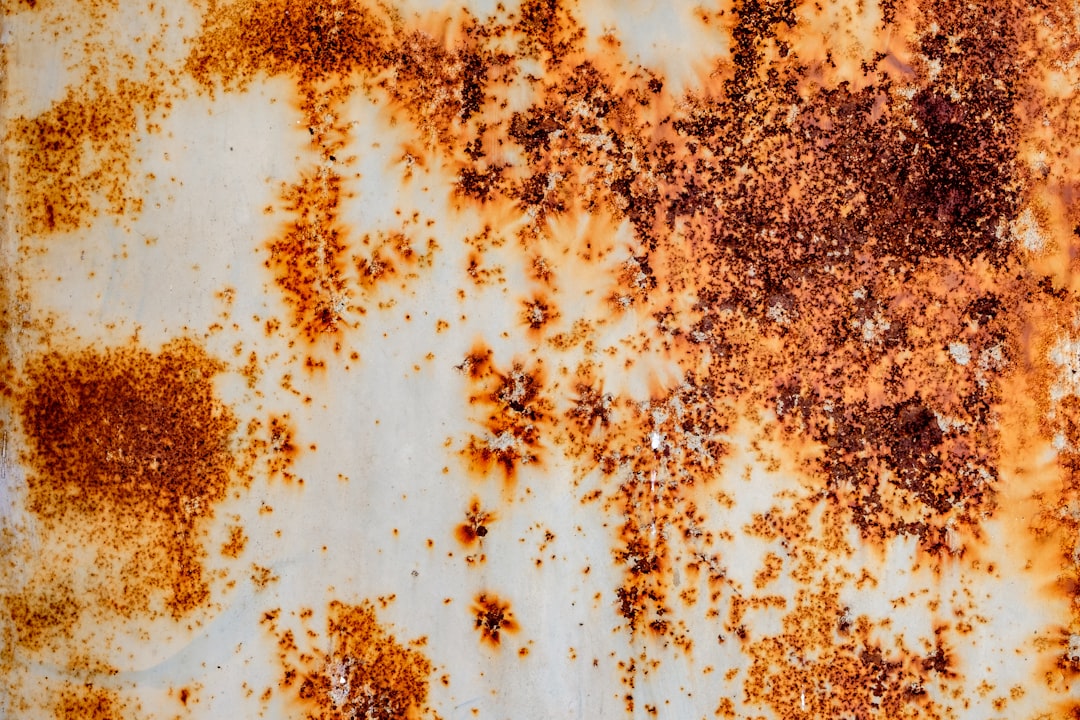
Research into the effects of temperature on corrosion has unearthed a worrying trend: a mere 15°C increase in temperature can lead to a 40% acceleration in corrosion rates, particularly affecting steel components commonly found in structures. This intensified corrosion is not merely a faster pace of degradation; it also suggests a disruption of the protective mechanisms that usually slow down the corrosion process in steel alloys. Given this strong influence of temperature on corrosion behavior, it is vital that those designing and building structures consider the potential impact of temperature fluctuations, especially in regions with varying climate conditions.
The issue is further compounded by the presence of dissolved solids in water, which we've already discussed as a major catalyst for corrosion in concrete structures. This combination of elevated temperatures and high concentrations of dissolved solids in the surrounding environment poses a double threat to the structural integrity of buildings and infrastructure. The result could be an accelerated rate of deterioration, unforeseen maintenance costs, and even potentially lead to compromises in structural safety. It's clear that there's a need for a more nuanced approach to understanding the complex interactions between temperature, dissolved solids, and corrosion to ensure long-term stability and structural safety of projects.
Research suggests that even a modest 15°C temperature increase can lead to a substantial 40% acceleration in the corrosion of steel reinforcement within concrete structures. This heightened sensitivity to temperature highlights the crucial role of environmental monitoring in regions prone to temperature fluctuations, especially when assessing long-term structural integrity.
It's also worth noting that the relationship between corrosion and the concentration of dissolved solids isn't a simple one-to-one correlation. Small changes in the total dissolved solids (TDS) of the surrounding water can lead to unexpectedly large increases in corrosion, hinting at much lower threshold levels for corrosion risks than previously understood. This finding may necessitate a re-evaluation of current safety margins for structures exposed to varied water chemistries.
Elevated temperatures not only directly accelerate corrosion but also increase the permeability of concrete. Warmer conditions may increase the size and connectivity of existing micro-cracks, potentially leading to faster water penetration and therefore, an even faster rate of corrosion than would otherwise occur.
At the interface between the steel and the concrete, fluctuations in pH and ion migration can lead to hydrogen embrittlement. This is a particularly concerning phenomenon as it can make the steel unexpectedly brittle without obvious signs of distress, raising the risk of sudden and catastrophic structural failures. It's a rather silent threat to infrastructure.
The presence of higher chloride ion concentrations, when coupled with rising temperatures, often exacerbates localized forms of corrosion like pitting. This localized corrosion often leads to much deeper and potentially damaging pits, as opposed to a more uniform degradation of the surface. Localized corrosion, in many cases, may be a larger concern in comparison to uniform corrosion.
The composition of the aggregates used in concrete plays a role in how readily ions like chlorides can migrate. Some aggregate types might inadvertently expedite ion transport, making a thoughtful selection of aggregates crucial during the material sourcing for concrete mixes. This emphasizes the interconnectedness of the corrosion problem and the overall composition of concrete.
The presence of micro-cracks, often created through thermal or mechanical stress in the concrete, can greatly accelerate the corrosion process by providing direct paths for corrosive agents. It appears that the behavior of micro-cracks may change with temperature, possibly leading to erratic and unpredictable rates of degradation.
When exposed to high TDS environments, differences in the types of metals within a structure can create localized electrochemical cells that accelerate corrosion. This galvanic corrosion, in essence, concentrates corrosion on less noble metals in contact with steel, potentially leading to a rapid decay of these more reactive metals.
Ion migration patterns are significantly influenced by various environmental factors, making accurate predictions of corrosion rates challenging. Changes in humidity and temperature can alter these patterns, potentially resulting in sudden and unexpected surges in corrosion activity.
Traditional non-destructive testing methods for structural health assessment often fall short when it comes to fully understanding the complex interplay of pH changes and ion movement near steel reinforcement. The difficulty in measuring these variables highlights the need for further refinement of monitoring techniques in order to ensure the long-term safety of structures. The complex dynamics that contribute to corrosion are often difficult to analyze using conventional means.
Structural Integrity Risks How Dissolved Solids in Water Accelerate Steel Reinforcement Corrosion in Concrete Foundations - Load Bearing Capacity Drops 25% After 5 Years of Chloride Exposure
Exposure to chloride ions can significantly weaken reinforced concrete structures over time. Research suggests that after only five years of chloride exposure, the load-bearing capacity of these structures can drop by a substantial 25%. This decline is primarily due to the rapid corrosion of the steel reinforcing bars embedded within the concrete. The corrosion process is amplified in areas of sustained stress and in the presence of cracks, which allow water and oxygen to readily reach the steel. This accelerated corrosion can lead to a variety of structural problems, including premature buckling and reduced flexibility of structural members, ultimately compromising the foundation’s ability to bear loads effectively. Recognizing the implications of these changes is important as it highlights the risk associated with aging infrastructure in environments where chloride exposure is a concern. It's becoming more apparent that proactive measures and careful assessments of structural integrity are increasingly vital for safeguarding our built environments, particularly as infrastructure ages and faces various environmental threats.
Researchers have found that after five years of exposure to chloride-rich environments, the load-bearing capacity of reinforced concrete can decrease by up to 25%. This significant reduction highlights the insidious nature of chloride-induced corrosion.
While concrete is intended to provide a protective layer for the reinforcing steel, the presence of chloride ions can alter that dynamic. Chloride ions are highly mobile and can penetrate concrete relatively quickly, often reaching the steel within a few years, particularly in environments with high concentrations of dissolved solids. The rate of chloride penetration is influenced by factors such as the concrete mix itself, the size and interconnectedness of microcracks, and the temperature of the environment.
The specific type of steel used in reinforcement also plays a critical role in how it handles chloride exposure. Stainless steel alloys designed for corrosive environments exhibit significantly greater resistance to chloride attack than standard mild steel. Understanding the inherent vulnerabilities of steel is essential for engineers selecting materials for structures that will be exposed to chloride-rich waters or environments where de-icing salts are used.
Moreover, temperature and the formation of corrosion products can modify the corrosion process. Rising temperatures increase the rate of corrosion and can change the solubility of various salts in the water, potentially leading to more corrosive conditions. Rust and other corrosion products also introduce changes in the local pH, further intensifying corrosion.
This reinforces the importance of taking a holistic view of the entire environment surrounding the concrete structure. The complex interactions between the type of steel, the chemistry of the concrete, the presence of microcracks, temperature, and the presence of dissolved solids can significantly influence the rate at which corrosion occurs.
Furthermore, repassivation, the steel's natural process of attempting to reform a protective oxide layer, can be severely compromised in chloride-rich environments. This means the steel may not be able to recover and protect itself from ongoing corrosion, significantly accelerating damage over time.
It's also noteworthy that even seemingly low levels of dissolved solids can still lead to corrosion. Research suggests a cyclic corrosion pattern is induced even by seemingly harmless amounts of dissolved solids, revealing a potential vulnerability in current design and maintenance practices. This means some structures assumed to be safe might still be susceptible to corrosion-induced degradation.
Localized corrosion, which includes pitting, is also a major concern, accounting for a substantial proportion of concrete structure failures. Localized forms of corrosion need to be factored into the design and inspection procedures rather than relying solely on average corrosion rates.
Finally, the interplay between humidity, temperature, and dissolved solids makes the assessment of long-term corrosion particularly challenging. Even small fluctuations in environmental conditions can influence the pace at which corrosion progresses. This underscores the need for detailed assessments of specific environmental conditions to ensure the long-term safety and integrity of structures exposed to aggressive environments.
The research on chloride-induced corrosion underscores the complexity of designing and maintaining durable concrete structures. It highlights the need for a more comprehensive approach to materials selection, design, and long-term monitoring of infrastructure, especially in environments where aggressive agents like chloride ions are present.
Structural Integrity Risks How Dissolved Solids in Water Accelerate Steel Reinforcement Corrosion in Concrete Foundations - Field Data From 2024 Bridge Inspections Maps Correlation Between TDS and Spalling
Recent bridge inspections conducted in 2024 have revealed a strong connection between the level of dissolved solids in water and the appearance of spalling on concrete bridge structures. Spalling, where concrete fragments break away from the surface, seems to be worsened by the quickening pace of corrosion in the steel reinforcement bars within the concrete. This heightened corrosion is especially evident in areas where the water has a high concentration of dissolved solids. Water and salts that are dissolved in it penetrate the concrete through tiny cracks, accelerating the deterioration process and creating major threats to the long-term strength and safety of bridges.
It is becoming clear that we need more effective monitoring and maintenance plans to deal with these corrosive environments and the way bridge structures deteriorate. It's essential to factor in this new data when evaluating bridge health to increase the lifespan and ensure the safety of our critical infrastructure. While the earlier sections emphasized the corrosive influence of dissolved solids, this new data helps further pinpoint specific issues and their connection to real-world problems. There's a need to explore and better understand the extent of damage caused by high TDS concentrations and find more precise ways to forecast the future integrity of bridges.
Field data gathered from the 2024 bridge inspections has revealed some intriguing connections between the concentration of total dissolved solids (TDS) in water and the occurrence of spalling. It seems that higher TDS levels correlate with specific patterns of concrete damage, hinting that certain bridge designs or materials might be more vulnerable to corrosion in environments with elevated TDS.
A closer examination of areas where spalling is evident shows that the underlying microstructure of the concrete degrades more quickly in high TDS environments compared to typical conditions. This leads to distinct and more severe deterioration processes. Interestingly, the bridge inspection data suggests that there are certain TDS thresholds that, when surpassed, lead to a rapid acceleration in spalling. This might prove a useful warning sign for older bridges operating in areas with water quality that varies.
The locations of corrosion-related failures, as observed in the inspection maps, are not randomly distributed. They seem to cluster in certain regions, implying a link to regional variations in water chemistry that engineers designing future structures should keep in mind. Electrochemical measurements taken during the inspections have unveiled that in areas with higher TDS, not only is corrosion faster but also the overall reactivity of the steel seems to be increased. This is more complex than just simple acceleration, and creates a challenge for predictive maintenance.
Surprisingly, spalling seems to occur more frequently in areas that were previously identified as having stress concentrations. This points to a potential synergistic effect where mechanical loading combined with the corrosive influence of high TDS could dramatically increase the risk of concrete failure. There's also evidence that the specific mix of concrete used can make a difference in how vulnerable a bridge is to corrosion-induced spalling. Some mixes, apparently, perform better in high-TDS settings than others.
Observing the inspection data over time, it's becoming clear that spalling begins to become a significant issue roughly three years after the initial exposure to high TDS. This is a delayed effect that can make maintenance planning challenging. Based on the 2024 inspections, we've also gained a better understanding of what spalling can indicate. Previously, it was often viewed as just a surface problem, but now we know it's a signal of much deeper problems affecting the structural integrity of the bridges.
The observed correlations between TDS and spalling are driving improvements in the development of predictive models for corrosion. These models now incorporate both environmental factors, like water quality, and structural characteristics, making it possible that we can design future bridges that are more resistant to the consequences of high-TDS environments. All of these insights suggest that using the lessons learned from these 2024 inspections could help improve the safety of bridges and other infrastructure in the future.
More Posts from aistructuralreview.com: