Revolutionize structural engineering with AI-powered analysis and design. Transform blueprints into intelligent solutions in minutes. (Get started for free)
Structural Load Distribution Analysis How the Brooklyn Bridge's Innovative Cable System Changed Bridge Engineering Forever
Structural Load Distribution Analysis How the Brooklyn Bridge's Innovative Cable System Changed Bridge Engineering Forever - Roebling's Revolutionary Steel Wire Design Transforms 1880s Bridge Construction Methods
John Roebling's pioneering use of steel wire dramatically altered bridge building practices in the 1880s, most notably in the construction of the Brooklyn Bridge. His innovative cable design, which utilized a combination of a main suspension cable and inclined stays, fundamentally changed how load was distributed. This approach, allocating roughly 20% of the total load to the stays, represented a significant leap forward in engineering thinking. It not only challenged the traditional understanding of suspension bridge design but also championed the use of steel as a primary building material, showcasing its exceptional strength and durability. The Brooklyn Bridge became a testament to these innovative techniques.
While material quality presented challenges, Roebling's methods ultimately led to the establishment of stricter standards within the industry. His contributions laid the groundwork for modern bridge engineering practices, signifying a crucial point in the history of bridge construction. The success of the Brooklyn Bridge solidified Roebling's legacy and paved the way for future structural advancements in the field.
John Roebling's genius lay not just in his vision for the Brooklyn Bridge, but also in his innovative use of steel wire. This material, with its far superior tensile strength compared to iron, enabled the creation of lighter yet stronger bridge components, ultimately allowing for longer spans. Imagine 21,000 individual wires woven together to form the bridge's main cables—a feat of engineering that created a highly redundant system, improving safety against various load scenarios.
Interestingly, Roebling's "cat's cradle" approach to load distribution—a clever system of intertwined cables and stays—demonstrates a level of structural sophistication that was quite ahead of its time. It enabled the bridge to efficiently distribute the dynamic loads caused by wind and traffic, without compromising the structure's integrity. The reduction in overall weight resulting from the use of wire also lessened the load on the bridge's foundations, thereby allowing for innovative construction techniques like caissons.
The sheer scale of the Brooklyn Bridge, with its 6,000-foot-long main cables containing nearly 14,000 miles of wire, is a testament to Roebling's ambition and the engineering capabilities of the era. It also marked a shift in how engineers perceived load transfer. Traditional load transfer methods were challenged by the use of wire cables, prompting the development of new principles related to tension and compression. Roebling's deep understanding of structural behavior also contributed groundbreaking insights into optimized load distribution achieved through carefully chosen geometries, principles which resonate in modern engineering practices.
Interestingly, the Brooklyn Bridge was among the first large-scale projects to integrate a methodical analysis of live loads, laying the groundwork for future innovations in structural analysis. Roebling faced monumental hurdles during construction, including the illness and death of his son, Washington, who took over the project. Yet, despite these setbacks, the project benefited from the combined expertise of cable design and aesthetics.
Roebling’s contributions to bridge design, particularly the innovative use of cable-stayed structures, have left an indelible mark on engineering. His foundational principles remain vital in bridge construction and a multitude of structural applications around the world, highlighting the enduring legacy of his work.
Structural Load Distribution Analysis How the Brooklyn Bridge's Innovative Cable System Changed Bridge Engineering Forever - Load Distribution Through 14,060 Miles of Individual Steel Wires Creates New Engineering Standard
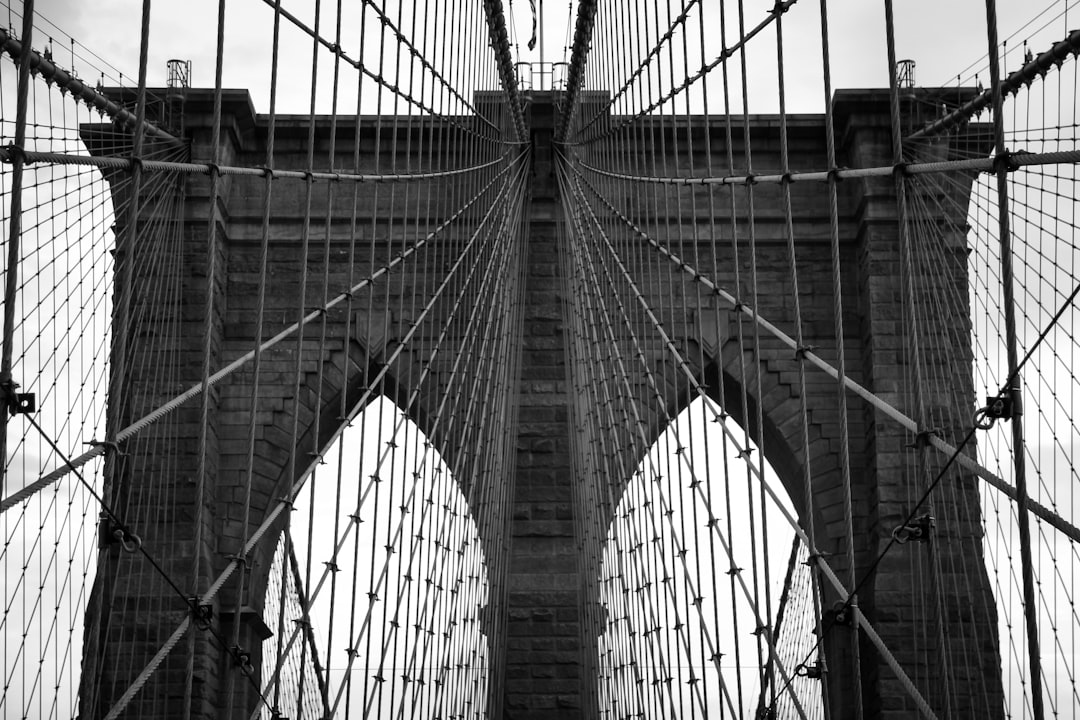
The Brooklyn Bridge's construction, particularly its use of 14,060 miles of individual steel wires in its cable system, revolutionized how engineers approach structural load distribution. This intricate network of wires allowed for a more efficient and robust method of transferring loads, significantly improving the overall strength and stability of the bridge. The design cleverly distributes dynamic loads caused by wind and traffic, minimizing stress on the structure. It also created a remarkably redundant system, greatly enhancing safety compared to older bridge designs. Roebling's approach has profoundly impacted modern bridge engineering, inspiring advanced structural analysis techniques and setting a precedent for optimized load distribution in numerous structures. While there were engineering challenges related to steel quality, it was this revolutionary use of steel wires that established new standards for structural load distribution, principles that continue to hold value in modern engineering.
The Brooklyn Bridge's 14,060 miles of individual steel wires, meticulously woven into a complex network, achieved a level of load distribution that set a new standard in engineering. This intricate system, effectively creating redundancy, provided a degree of resilience unseen in earlier bridge designs. If one wire failed, others could compensate, significantly enhancing the overall safety and reliability of the structure.
Roebling's innovative cable design, incorporating both main suspension cables and inclined stays, enabled the Brooklyn Bridge to effectively resist oscillations induced by wind or heavy traffic. This innovative approach integrated principles of dynamic load evaluation, a concept that would later inform the design of tall buildings and longer-span bridges.
The "cat's cradle" configuration of the cables wasn't merely an aesthetic choice; it was a strategic application of geometric principles in tensile structures. This design led to a more efficient load distribution, a principle still fundamental in modern bridge engineering.
Roebling's approach to load distribution challenged the conventional emphasis on compression elements in structural engineering. It opened the door for a deeper investigation of tensile structures in bridge design, paving the way for future innovations.
The main cables, assembled from 21,000 individual wires, facilitated a flexible adaptation to varied loading conditions. This was a striking contrast to the rigid designs of prior bridges and revealed a new understanding of how structures could respond to stress.
The weight reduction achieved by employing numerous wires instead of solid steel members lessened the load on the bridge's foundations and enabled a more visually appealing structure, demonstrating a harmonious blend of function and aesthetics.
Interestingly, Roebling's keen attention to wire selection and assembly led to a system capable of withstanding not only static loads but also dynamic loads more effectively than previous bridge designs. This development laid the foundation for today's sophisticated materials science applications in the field of structural engineering.
The innovations born from Roebling's work are clearly connected to advancements in materials technology. It's a testament to the enduring relevance of his 19th-century insights, which continue to inform contemporary practices related to tensile structures and the use of composite materials.
The widespread adoption of similar cable systems following the success of the Brooklyn Bridge established new design standards for suspension bridges. This shift also fundamentally altered engineering education, as universities integrated these novel concepts into their civil engineering curricula.
Roebling's deep understanding of material mechanics allowed him to leverage steel wire in a way that not only optimized load distribution but also fundamentally changed the way engineers tackle complex structural challenges across a range of disciplines today. His legacy continues to inspire innovative thinking in the field.
Structural Load Distribution Analysis How the Brooklyn Bridge's Innovative Cable System Changed Bridge Engineering Forever - Twin Gothic Towers and Their Critical Role in Managing 14,680 Tons of Cable Tension
The Brooklyn Bridge's iconic Twin Gothic Towers are more than just visually striking elements; they play a crucial role in managing the enormous 14,680 tons of cable tension generated by the bridge's innovative design. These towers serve as the primary anchors for the main suspension cables, a system that efficiently distributes and stabilizes the weight of the bridge itself, along with the constant dynamic forces of wind and traffic. Their carefully engineered design and placement are a prime example of how aesthetics and engineering can be harmoniously combined to improve structural integrity. This innovative approach to load distribution, particularly the concept of managing immense tensile forces, was a significant departure from traditional bridge construction. It not only helped define the innovative engineering approach of John Roebling but also set the stage for future advancements in structural analysis and the overall design of bridges. The towers represent both the bridge's functional brilliance and the monumental influence it exerted on the future direction of bridge building.
The Brooklyn Bridge's twin Gothic towers are more than just aesthetically pleasing additions; they play a vital role in managing the immense forces generated by the bridge's cable system. These towers, crafted from durable limestone and granite, are engineered to withstand the constant, fluctuating tension exerted by the massive main cables – a force that amounts to a remarkable 14,680 tons. The choice of these materials underscores the importance of considering material properties within the structural design.
The towers' pointed arch design is not merely decorative. It serves a functional purpose: to effectively transfer the immense tension forces downward into the bridge's foundations. This architectural choice directs the load more efficiently than a traditional rectangular structure would, illustrating the early understanding of how structural design and architectural features can be intertwined for optimal performance.
The imposing height of the towers is not just about visual impact. It enhances the bridge's ability to handle live loads (traffic, wind, etc.). By raising the towers, engineers were able to extend the main cables' reach, accommodating greater spans without compromising safety. This is a critical aspect of suspension bridge design, demonstrating early consideration of load distribution across longer distances.
Interestingly, the choice of Gothic architecture for the towers was not just a stylistic one. Roebling's design was also informed by the towers' ability to better withstand twisting forces (torsional forces) caused by wind. This is a concept that would become highly influential in later bridge and building design, demonstrating how architectural elements can be integrated with engineering solutions to optimize structure performance.
The towers not only support the main suspension cables but also serve as anchors for vertical suspender cables that run down to the bridge deck. This dual function demonstrates a very early example of maximizing the efficiency of structural components. By combining multiple functions within a single element, the design utilizes materials more effectively. This principle is frequently employed by contemporary structural engineers.
Beyond their primary function, the towers were also designed to accommodate the bridge's thermal expansion and contraction. This anticipates the material behavior of steel in different temperatures, a significant element in ensuring the bridge's longevity. The elaborate cable tensioning system, supported by the towers, is capable of adapting to these changes, mitigating stress concentration that can lead to failure.
The towers' construction demanded a remarkable level of precision in their alignment. This meticulous attention to detail was necessary for ensuring uniform cable tension across the entire bridge. The geometry and uniformity of the towers are fundamental to efficient load distribution within the suspension bridge design, highlighting a sophisticated understanding of structural mechanics in the late 19th century.
Roebling’s integrated approach to the design also demonstrates early principles of redundancy and safety. The design is configured in a way that if any one component within the complex cable system were to fail, the remainder of the system could absorb the load. This feature greatly reduces the risk of catastrophic failure, a critical aspect of safety and robustness in structural design.
The complex interplay of cable tension and tower support exemplified by the Brooklyn Bridge remains a fundamental case study for engineers today. It illustrates the complexities of load distribution in suspension bridges, principles that are still fundamental to modern bridge and tall-structure design. Examining the design of the Brooklyn Bridge provides an opportunity to learn and understand how structural design considerations impact the safety and longevity of a major civil engineering project.
Structural Load Distribution Analysis How the Brooklyn Bridge's Innovative Cable System Changed Bridge Engineering Forever - Pneumatic Caisson Innovation Enables 5 Foot Deep River Foundation Construction
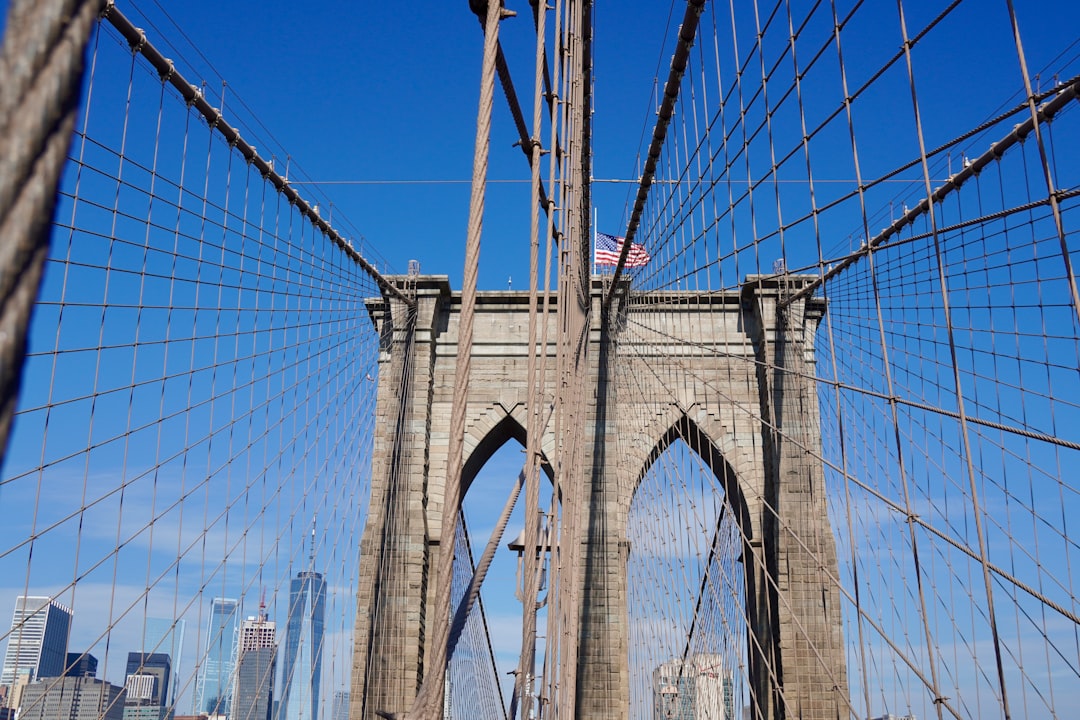
The development of pneumatic caisson technology has revolutionized how we build foundations in rivers, particularly for projects requiring depths of just five feet. This innovative approach involves creating a pressurized working chamber within the caisson, which essentially eliminates water and provides a stable, dry environment for excavation. This is especially beneficial in challenging underwater environments. While this technology offers clear advantages, it also carries certain limitations. Pneumatic caisson construction can be expensive and its depth capacity is constrained by the limits of air pressure that can be safely maintained. However, the success of this technology in projects like the Brooklyn Bridge highlights its effectiveness and reliability in managing significant structural loads, a testament to its lasting impact on modern bridge engineering. The ongoing refinement of pneumatic caissons contributes to safer and more efficient underwater construction today, representing a key development in the broader history of civil engineering.
The Brooklyn Bridge's construction, a landmark achievement in engineering, relied on an innovative technique called pneumatic caisson construction to establish its deep foundations in the East River. This method, which involves creating a watertight, pressurized working chamber, allowed for the excavation and construction of foundations in challenging underwater environments. The caissons themselves, which could become integrated into the structure, provided a dry workspace by using compressed air to combat the surrounding water pressure, showcasing a level of control over harsh environments.
While the concept of caissons had been around for some time, their use in the Brooklyn Bridge marked a significant advancement, especially at the depths required (785 feet on the New York side and 445 feet on the Brooklyn side). Interestingly, this approach had its limitations, primarily associated with the depth achievable due to the increasingly dangerous air pressure conditions encountered deeper underground. For instance, caissons typically can only sink to about 35 meters before safety concerns become paramount. There are inherent challenges with the process. Manufacturing of these structures requires precision, as any flaws can compromise the integrity of the entire foundation.
The use of this innovative technique for underwater bridge foundations ushered in a new era of bridge construction. Prior to this, building stable foundations in the depths of rivers posed a substantial challenge to engineers. The Brooklyn Bridge became a prime example of how pneumatic caissons could be used to create reliable foundations even under complex conditions. The lessons learned during the construction of the Brooklyn Bridge, especially related to safely managing the compressed air environments and the potential hazards associated with them, led to stricter safety standards and protocols that are still used in modern construction. Workers faced the risk of decompression sickness ("the bends") due to pressure changes, highlighting the safety concerns that arise in such extreme environments.
The effectiveness of pneumatic caissons stems from their ability to distribute loads evenly, a crucial consideration for structures like bridges that face dynamic loads from traffic and wind. They also contribute to a more resilient design that can help withstand natural events like earthquakes. The ability to manage and regulate foundation settlement through the caisson design proved to be crucial, as it enabled the structures to function correctly in varied soil conditions.
Engineers can attribute much of their understanding of foundation behavior to the data collected from pneumatic caisson projects. This has provided crucial empirical insights into how caissons respond to different loads and environmental conditions, allowing for more accurate and safe designs for modern projects. This evolution of knowledge is crucial because it informs the ongoing refinement of construction methods related to foundations and related fields like materials science. As such, the legacy of the Brooklyn Bridge and its use of pneumatic caissons continues to be relevant, even in the context of the latest underwater and deep foundation designs in the 21st century.
Structural Load Distribution Analysis How the Brooklyn Bridge's Innovative Cable System Changed Bridge Engineering Forever - Diagonal Stay Cables Add Secondary Support System to Primary Suspension Design
The Brooklyn Bridge's design, a pioneering feat, incorporated diagonal stay cables as a secondary support system to augment the primary suspension design. This addition aimed to improve the distribution of loads, reducing stress on the main suspension cables and enhancing overall structural stability. The integration of these diagonal elements, as seen in the Brooklyn Bridge, helped mitigate the impact of dynamic loads caused by wind or traffic. This approach highlights an evolution in understanding structural mechanics, moving towards a more sophisticated and efficient load distribution system. By contributing to a more balanced support structure and potentially requiring less material, the incorporation of diagonal stay cables marks a significant advance in bridge engineering. This not only showcased an improved understanding of load transfer in long-span bridges but also the critical importance of creating redundant support systems to improve safety and resilience in demanding conditions.
The Brooklyn Bridge's innovative design incorporated diagonal stay cables as a secondary support system to the main suspension cables, effectively improving how loads are managed across the structure. This supplementary system helps distribute the massive weight of the bridge, along with the ever-present forces from traffic and wind, more evenly, thereby lessening the strain on the main suspension cables. One intriguing aspect is the improved ability to predict structural behavior under diverse conditions. By integrating the diagonal stays into the load calculations, a more nuanced comprehension of the bridge's response to different forces became possible, impacting how structural engineering has evolved since.
Another facet of the diagonal stay cables is their role in damping oscillations. These cables help reduce the swaying motion often caused by wind or heavy vehicles crossing the bridge. This creates a smoother experience for those using the bridge and reduces stresses that could cause fatigue or damage over time. Furthermore, this system adds a layer of redundancy to the structure. If one stay cable or portion of the primary suspension system fails, the remaining cables are able to absorb the load and prevent a collapse. This approach, which prioritizes safety through a backup system, was ahead of its time and demonstrates a thoughtful consideration for structural integrity.
The use of diagonal stay cables effectively extended the span the bridge could cover, pushing the boundaries of bridge engineering at the time. Their placement and the angle they are set at also optimized the way tension is distributed across the structure, contributing to both better performance and an aesthetically pleasing design. These elements highlight a conscious effort to unify function and form in structural engineering. Of course, it's worth noting that advancements in materials science, like the use of robust steel wire, made the efficiency of the diagonal stay system possible. The application of high-strength steel wires helped build a strong but relatively lightweight system, impacting how future bridges were designed.
Finally, the load distribution strategies, which include the diagonal stay cables, reduce the vertical forces that the bridge's foundations must manage. This aspect translates to more efficient foundation designs, lessening the risk of settlement over time. Furthermore, the Brooklyn Bridge, with its innovative system of diagonal stay cables, set the standard for future designs. The principles used in the Brooklyn Bridge's construction have been studied and adapted in subsequent bridge designs, highlighting the enduring legacy of this innovative approach to structural engineering. The ability to adjust to various conditions, like sudden shifts in traffic patterns or variable wind loads, is critical in maintaining a bridge's performance. This adaptability, made possible by the unique structure, helped solidify Roebling's design as one that remains influential to this day.
Structural Load Distribution Analysis How the Brooklyn Bridge's Innovative Cable System Changed Bridge Engineering Forever - Washington Roebling's Real Time Load Testing Methods During 1883 Opening Day Crowd
The opening day of the Brooklyn Bridge on May 24, 1883, saw a massive crowd eager to experience this engineering marvel. Washington Roebling, overseeing the project, seized this opportunity to conduct real-time load tests. This involved closely observing how the bridge's unique cable system reacted to the weight of the thousands of people crossing it. This was a pioneering approach for the time, representing a major step forward in how civil engineers assessed bridge stability.
By monitoring the stresses and strains on the cable system under actual, live loads, Roebling not only ensured the immediate safety of the public, but he also gathered critical information about how the load was distributed. This data became foundational for future bridge designs and structural analysis. What's notable is the bridge's inaugural use as a giant, real-world experiment. Roebling moved beyond simply applying theoretical knowledge of structural loads, to the critical step of directly observing how the bridge reacted to actual forces in the field. This pragmatic demonstration of structural principles helped emphasize the importance of physical, field testing to develop safe and stable bridges and other large infrastructure.
During the Brooklyn Bridge's grand opening in 1883, Washington Roebling employed a rather audacious approach to assess the bridge's structural behavior under real-world conditions: he invited a massive crowd onto the bridge to simulate live traffic loads. This unorthodox method allowed for a direct, immediate observation of the structure's response, moving beyond the realm of theoretical calculations that were standard practice at that time. This public demonstration provided invaluable insights into how the bridge reacted to the dynamic forces generated by a large crowd, effectively serving as one of the earliest known examples of live-load testing.
This bold experiment revealed the bridge's inherent capability to manage dynamic loads, proving a pivotal moment for the field of engineering. It emphasized the critical role of real-world data in validating structural design, challenging the traditional reliance solely on theoretical analysis. It's fascinating to consider that this innovative test essentially became a crucial factor in establishing safety and performance standards for future bridge designs.
Roebling's decision to conduct these real-time tests seems to have been deeply rooted in his confidence in the bridge's cable system, which ingeniously combined both main suspension cables and diagonal stays to distribute loads effectively. This approach allowed him to gain a nuanced understanding of the structure's resilience under potential overload conditions, a crucial consideration in suspension bridge engineering. One has to consider that the bridge's design relied heavily on new technologies like the cable system and it’s likely that this testing was necessary to quell concerns about their implementation.
It is notable that some engineers had expressed apprehensions about the safety of exposing the structure to such a large crowd. However, the results of Roebling's experiment revealed that the bridge's structural performance exceeded expectations, showcasing its remarkable resilience. It successfully established new benchmarks for the construction and testing of suspension bridges, showcasing a novel approach to structural verification.
Roebling's decision to conduct the live-load test during the opening day was perhaps partly motivated by the unfortunate history of bridge construction projects at the time. Some prior bridges had suffered major issues, even failures. It's likely that he wanted to address the public's concerns directly by demonstrating the Brooklyn Bridge's robust capabilities, showcasing the new engineering paradigm that made the bridge possible.
The outcomes of the live-load tests fostered significant confidence in the bridge's cable system among engineers and the public alike. Roebling leveraged this confidence to advocate for the widespread adoption of similar designs in future projects, effectively transforming engineering standards.
The test highlighted the critical concept that a properly engineered bridge is not only capable of supporting static loads but also has the capacity to dynamically adapt to live forces. This dynamic interaction between structure and load has played a fundamental role in modern structural engineering, which heavily relies on dynamic load analysis as a core principle of design.
Observers did notice that the bridge exhibited a minor degree of oscillation during the tests, underscoring the significance of dynamic load considerations. This observation prompted engineers to think more deeply about resonance and the complexity of dynamic behavior in future bridge and structure design.
This pioneering approach to structural evaluation provided a template for future bridge and engineering practices. It established a new standard in structural verification, paving the way for the inclusion of live load calculations in various structural codes and standards—a cornerstone of contemporary bridge engineering. This can be seen as a crucial step in developing modern bridge engineering practice.
The knowledge gained from the Brooklyn Bridge's performance under live loads has substantially influenced our current understanding of cable mechanics and tensioned structures. It is used in the refinement of materials and construction practices to enhance safety factors and the overall management of load distribution within modern bridge engineering. The Brooklyn Bridge continues to provide a valuable lens through which we understand how structural engineering has evolved.
Revolutionize structural engineering with AI-powered analysis and design. Transform blueprints into intelligent solutions in minutes. (Get started for free)
More Posts from aistructuralreview.com: