The Roebling Legacy Engineering Marvels Behind the Brooklyn Bridge's Construction
The Roebling Legacy Engineering Marvels Behind the Brooklyn Bridge's Construction - John Augustus Roebling's Revolutionary Wire Rope Suspension Design
John Augustus Roebling's groundbreaking work with wire rope revolutionized bridge design. By pioneering the use of this material, he enabled the creation of bridges that were not only longer but also more robust than ever before. His initial experiments, notably in Pittsburgh, were instrumental in shaping his vision for the Brooklyn Bridge. This iconic structure stands as a testament to the practicality and durability of Roebling's revolutionary approach. While facing substantial hurdles during the bridge's construction, Roebling's ingenious methods set a precedent for the future evolution of bridge engineering. The impact of his work transcended the Brooklyn Bridge, shaping the designs of other major structures and establishing a new standard for bridge building. This impact showcases not only his impressive technical skills but also his capacity to reimagine the possibilities within civil engineering.
John A. Roebling's approach to wire rope design was a significant leap forward. Instead of relying on traditional solid metal bars, he ingeniously employed numerous smaller wires twisted together to form strands and then cables. This technique dramatically increased tensile strength while reducing the overall weight of the structural elements. This was particularly crucial for suspension bridges, where minimizing weight while maximizing strength is essential.
The flexibility introduced by this innovative approach was another crucial advantage. The ability of the wire rope to absorb dynamic loads—both from wind and the movement of traffic—became a vital element in ensuring long-term stability and durability. This ability to adapt to changing loads proved to be key in creating resilient bridges.
Furthermore, the composite nature of the wire ropes – built from many smaller elements—inherently provided resistance to fatigue. By allowing individual wires to flex independently, the risk of catastrophic failure due to cracking or deformation was significantly reduced. This design feature also contributed to the longevity of the structures Roebling envisioned.
Roebling's manufacturing process, which utilized the technique of "stranding," also proved advantageous. Twisting the wires into strands before assembling them into cables effectively increased the cable's surface area. This enabled a stronger and more secure grip on the supporting structures, thus enhancing the overall integrity of the bridge designs.
Interestingly, Roebling incorporated a considerable safety margin into his designs. He deliberately over-engineered his structures, incorporating factors of safety often exceeding two. This conservative approach—uncommon at the time—was a calculated decision to ensure the bridges could withstand forces well beyond what was anticipated during their intended lifespan. This element of design was indicative of a careful and methodical approach.
The choice of materials for his wire ropes was also carefully considered. High-carbon steel, with its excellent strength-to-weight ratio, became the favored material. This choice played a critical role in achieving optimal performance while minimizing structural weight, which was particularly advantageous for suspension bridges.
Roebling's anchorage system itself was cleverly designed, leveraging the weight of the bridge's elements to counter tensile forces. This ingenious approach resulted in a simpler, less complex design, reducing the need for excessive engineering to manage extreme loads. His clever use of gravity can be considered a stroke of genius.
Prior to construction, Roebling meticulously tested the performance of his wire rope designs. Through rigorous experimentation, he measured the ropes' breaking strength and elasticity. The data collected during these experiments provided critical insights that shaped the final design parameters, reducing guesswork and enhancing safety.
Roebling understood the importance of considering deformation in his structural models. In an era where this was less common practice, he proactively integrated calculated deformation into his bridge designs. This approach led to more predictable behavior under stress, resulting in a level of sophistication rarely seen in suspension bridge design at the time.
The introduction of this new technology was not without challenges. Throughout the initial planning stages of the Brooklyn Bridge, Roebling faced considerable skepticism regarding the efficacy of his wire rope approach. Several established engineers questioned whether his design would withstand the anticipated loads. However, Roebling's insistence on rigorous testing and demonstration eventually allayed these doubts and helped pave the way for the construction of his iconic bridge.
The Roebling Legacy Engineering Marvels Behind the Brooklyn Bridge's Construction - Pneumatic Caissons The Foundation of Brooklyn Bridge's Strength
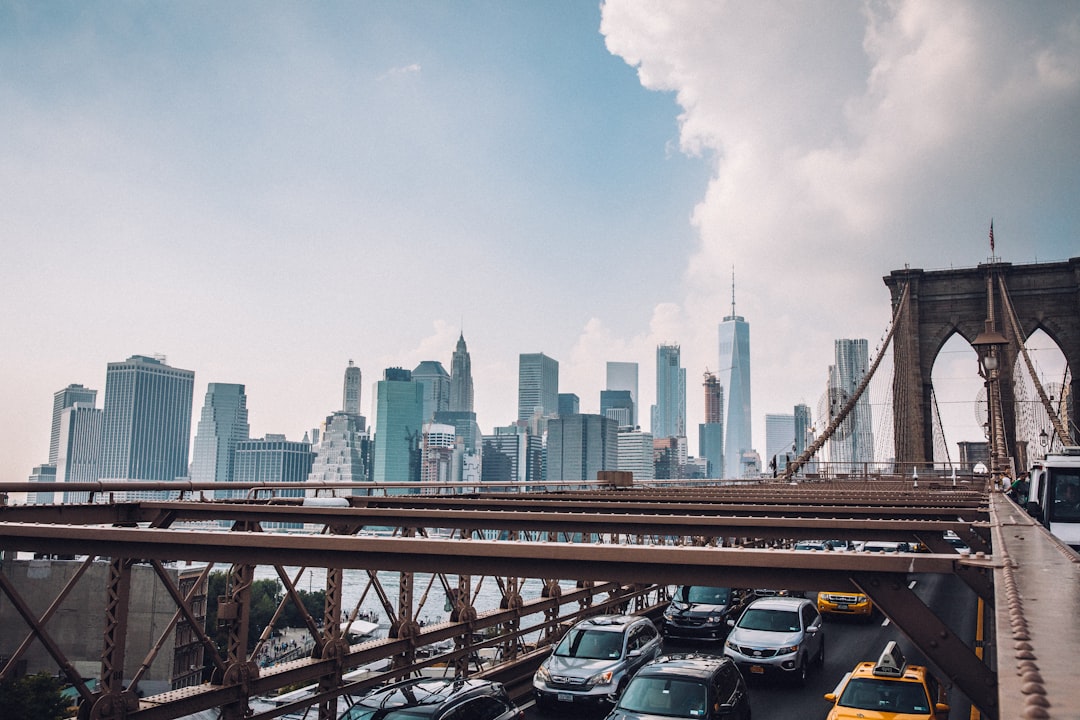
The Brooklyn Bridge's construction, particularly its foundation, relied heavily on a novel approach for the time: pneumatic caissons. These structures, essentially sealed chambers, were pressurized with air to push back the water and soil, allowing workers to excavate and lay foundations beneath the East River. This was a remarkable innovation, enabling engineers to build in a way previously impossible in underwater environments.
The caissons themselves were equipped with airlocks, essential for managing the pressure difference between the outside and the internal working space. This was a critical safety feature, preventing sudden pressure changes that could cause flooding or endanger the workers. But these structures were immense, made of robust cast iron or steel, capable of enduring the enormous pressure exerted by the surrounding water, which could be as high as five atmospheres at the depth of the bridge's tower foundations.
Despite the remarkable ability of the caissons to allow for work in the challenging environment, there was a downside. Workers faced a previously unseen risk, referred to as "caisson disease" or "the bends." This ailment was a result of the pressure changes experienced when surfacing too quickly after extended periods within the pressurized caisson. It underscores the significant safety concerns inherent in this pioneering construction method.
The benefit of the caisson method was that construction could continue regardless of tidal changes. Unlike older methods, engineers could work continuously to excavate and establish a solid foundation. The caissons, weighing hundreds of tons each, were meticulously designed and shaped for gradual sinking, ensuring that they remained stable and correctly positioned. The challenge was the maintenance of air quality within the enclosed space. Compressed air, which is crucial for the caisson's functioning, could accumulate carbon dioxide and other potentially harmful contaminants. This necessitated complex and innovative ventilation systems to ensure a safe and tolerable environment for workers.
While innovative and successful, the use of pneumatic caissons did not come without its detractors. Some engineers of the time questioned the viability of these complex structures and their associated risks, preferring more traditional techniques. However, the success of the Brooklyn Bridge construction, in which caissons played a crucial role, helped establish this methodology as a standard in future underwater projects. This innovative approach significantly influenced the construction of tunnels and various structures worldwide.
Curiously, the deep excavations carried out using the pneumatic caissons unearthed relics from New York's earliest settlements. This unintended outcome provided invaluable insights into the region's history prior to the bridge. It serves as a poignant reminder that construction projects, even those at the forefront of innovation, can sometimes unearth the past, blending the new with the old in a powerful way. The Brooklyn Bridge stands as a testament to both engineering innovation and our deeper understanding of historical contexts, much of which came to light as a byproduct of the novel methods used in the construction process.
The Roebling Legacy Engineering Marvels Behind the Brooklyn Bridge's Construction - Washington Roebling's Innovative Use of Steel-Wire Cables
Washington Roebling's innovative approach to utilizing steel-wire cables was a crucial factor in the Brooklyn Bridge's success. Following his father's legacy, Washington took the reins and meticulously managed the creation of the bridge's colossal cables. Each cable was a marvel of engineering, consisting of 286 individual wires bundled together into strands, then further combined to form the final cable. This unique approach, employing numerous smaller wires, provided a significant increase in tensile strength while simultaneously introducing greater flexibility. This flexibility proved essential in allowing the bridge to better withstand the dynamic forces of wind and traffic, ensuring its long-term stability and durability. While facing considerable personal health complications during the bridge's construction, Washington Roebling's determination and innovative engineering skills ultimately led to a structure that profoundly impacted bridge design. The Brooklyn Bridge not only redefined bridge construction but also served as a springboard for future advancements in civil engineering. The lasting influence of his methods is evident in modern infrastructure, a testament to the enduring impact of his work.
The Brooklyn Bridge, a marvel of engineering completed in 1883, owes much of its success to Washington Roebling's innovative use of steel-wire cables. Taking over the project after his father, John A. Roebling's death, Washington played a crucial role in realizing his father's vision. John A. Roebling's revolutionary method of weaving steel wires into cables was fundamental to the bridge's design, providing a far stronger and more durable structure than previous iron bridges.
This novel approach involved spinning the cables on-site using traveling wire-winding carriages, a process that consumed over 18 months. Each cable is a composite of 286 individual wires, meticulously bundled into 19 strands and then wrapped together. It's quite remarkable that each of these massive cables, weighing almost seven million pounds, is formed from individual wires each weighing a substantial 1,200 pounds.
Roebling's choice of materials was key. High-carbon steel, with its impressive strength-to-weight ratio, proved superior to conventional iron. This allowed for both strength and a reduction in overall structural weight, a critical factor in a suspension bridge design. Interestingly, the cables exhibited a tensile strength averaging 100,000 psi, setting a new industry benchmark. The strength, coupled with the flexibility of the cable design, enabled the bridge to handle dynamic forces from wind and traffic effectively. This is where the inherent nature of the cable's construction – from many smaller wires – proved advantageous. By allowing for independent movement of individual wires, it greatly enhanced resistance to fatigue and reduced the likelihood of a catastrophic failure at a single point.
Before construction, extensive testing was performed on the wire rope, assessing breaking strength and elasticity. This empirical approach influenced design decisions, improving safety and reducing reliance on speculation. The process of “stranding” the wires before assembling the cables increased the cable's surface area, resulting in a more secure connection to the support structures. It is a sign of a detail-oriented and conservative mind that Roebling incorporated safety factors greater than 2 into his calculations, going above and beyond the conventional practice of the era. He also cleverly leveraged gravity by designing the anchorage system in a way that relied on the bridge's weight to counteract tensile forces, resulting in a simpler and less complex structure.
It is a testament to Roebling's dedication that, despite initial skepticism from engineers about the viability of the wire-rope design, he insisted on rigorous testing to validate his approach. His perseverance in overcoming doubts speaks to the innovative spirit often required for engineering breakthroughs. It's a fascinating reminder that progress rarely happens without some friction and resistance. In the end, Washington Roebling successfully navigated the complexities of the project and his father's legacy, demonstrating an ability to innovate while honoring the core principles of the original vision. His contribution remains an important part of the Brooklyn Bridge's engineering marvel, and it continues to influence bridge design and construction today. The Brooklyn Bridge, spanning 5,989 feet—the longest suspension bridge of its time—stands as a lasting tribute to Roebling's innovative spirit and the enduring power of his design.
The Roebling Legacy Engineering Marvels Behind the Brooklyn Bridge's Construction - Emily Warren Roebling's Crucial Role in Project Management
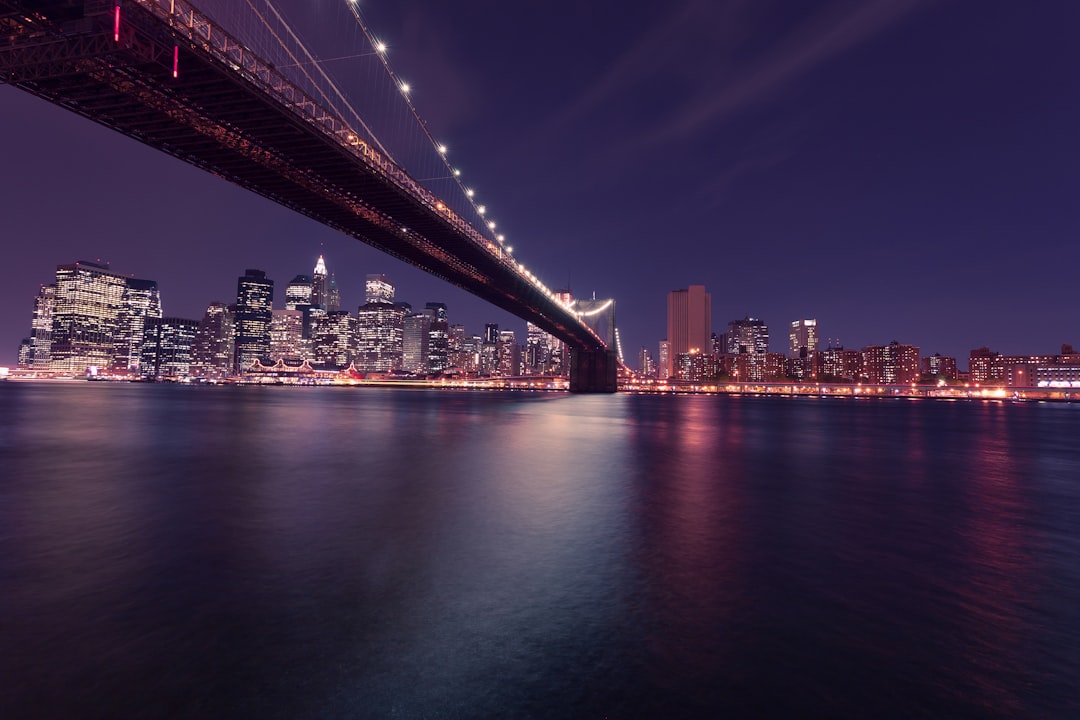
Emily Warren Roebling played a crucial role in the Brooklyn Bridge's construction, stepping in when her husband, Washington, fell ill and could no longer oversee the project. Her background, shaped by exposure to engineering through her husband and father-in-law, John A. Roebling, prepared her to tackle the challenges ahead. In a time when women's roles in engineering were severely limited, Emily's involvement was groundbreaking. She essentially became the project's field engineer, managing communications and coordinating with the workforce and other engineers to maintain momentum. This wasn't just a supervisory role; she actively used her engineering knowledge to keep the bridge's construction moving forward. Emily's actions were a powerful statement regarding the abilities of women in a field previously dominated by men. Her efforts were integral to the bridge's completion in 1883, a testament to her crucial management abilities. This accomplishment solidifies Emily's place not only within the context of the Brooklyn Bridge but also as a symbol of women's potential in engineering and project management. Her example has significance for both the field of engineering and the broader social movement advocating for women's equal access to professional endeavors.
Emily Warren Roebling's story during the Brooklyn Bridge's construction is a fascinating example of how individuals can step into unforeseen roles and make critical contributions to large-scale engineering projects. Initially, Emily's involvement wasn't planned, but after her husband, Washington Roebling, contracted a debilitating illness, she took on an unexpectedly central role. Despite having no formal engineering education, Emily quickly immersed herself in the intricacies of the bridge's construction. She avidly studied design documents and engaged with the engineers, revealing a rare aptitude for grasping complex technical concepts.
One of her key contributions was acting as the bridge between Washington, who was often confined to his home due to illness, and the construction crew. Employing a combination of letters, detailed project updates, and personal interactions, she ensured a steady flow of critical information across the barriers of distance and health. This role necessitated exceptional communication skills, as she was essentially the project's nerve center, conveying instructions and managing expectations. It's also worth considering the importance of her safety advocacy. Witnessing the inherently dangerous nature of the work, she was a strong voice for worker safety and helped promote the use of safety devices during an era where such matters weren't always prioritized.
Further, Emily's role extended to financial and contractual matters. She oversaw significant project funds and navigated the complexities of contracts and negotiations, demonstrating astute management abilities and an impressive ability to handle monetary responsibilities. In a time when women’s roles were severely limited, she was a powerful negotiator, a skilled communicator, and a competent manager. She skillfully fostered relationships with contractors, investors, and workers, ensuring that the project proceeded despite various hurdles. This highlights her ability to build consensus and maintain project momentum in a challenging environment.
Emily's story also reflects remarkable resilience in the face of societal bias. The idea of a woman acting as a project leader in a complex engineering endeavor was unheard of in that era. Yet, she steadfastly pursued her role, effectively acting as the project's surrogate chief engineer. It's fascinating to consider her unique position as a bridge between the technical side of the project and the larger social context. It's also notable that she diligently documented the project in her letters and reports, leaving a rich historical record of the process and providing a window into the challenges and triumphs faced by the project team.
Following the bridge's completion, Emily advocated for recognition of her own role and her husband's enduring contributions. Her legacy extended beyond the Brooklyn Bridge's physical structure. By breaking gender norms and taking the reins of this significant undertaking, she provided an inspiring example of what women could achieve in engineering. In essence, she served as a pioneer, creating a path for future generations of women to follow in her footsteps. Emily's example is not simply a historical anecdote; it is a beacon for those who seek to overcome limitations and challenge the traditional definition of roles in fields like engineering and project management. Her story reminds us that valuable contributions can emerge from unexpected sources, and that limitations can often be overcome through skill, determination, and a genuine commitment to the task at hand.
The Roebling Legacy Engineering Marvels Behind the Brooklyn Bridge's Construction - Overcoming Structural Challenges During 15-Year Construction
The 15-year construction of the Brooklyn Bridge was a saga of overcoming formidable structural challenges, a testament to the ingenious engineering pioneered by John A. Roebling and his successors. The project's path was not without obstacles; financial difficulties and the disruption of the Civil War threatened to derail the ambitious vision of a suspension bridge spanning the East River. Yet, through innovative solutions such as the use of pneumatic caissons and advanced steel-wire cable technology, the project steadily progressed. These solutions not only addressed the immense physical challenges of building within the East River but also solved complex logistical problems. Roebling's relentless drive was crucial in overcoming the doubts of established engineers who questioned the feasibility of his designs. His unwavering focus helped push the boundaries of traditional bridge building. The final realization of the Brooklyn Bridge was a monumental accomplishment, not only fulfilling Roebling's aspirations but also establishing new standards in engineering that profoundly impacted the development of suspension bridges globally. The bridge stands as a potent reminder of the triumph of human ingenuity over adversity, a lasting symbol of innovation permanently etched into architectural history.
The Brooklyn Bridge's construction, a monumental undertaking, was not without its share of structural challenges. The innovative use of pneumatic caissons, for instance, while enabling the creation of deep underwater foundations, also presented the significant challenge of managing high air pressures within the working chambers. These pressures, exceeding five atmospheres at certain depths, required meticulous engineering to maintain worker safety. Further, the technology used to manage pressure evolved throughout the project, revealing the adaptability of the engineers facing unforeseen circumstances.
The decision to utilize high-carbon steel, a material offering superior tensile strength compared to the then-common iron, introduced its own set of complications. Its tendency toward brittleness under specific conditions demanded a meticulous approach to quality control, preventing potential structural failures. It's notable how materials science limitations impacted the construction process.
The skepticism surrounding Roebling's innovative wire rope design was addressed through extensive testing of individual wire strengths. Washington Roebling, carrying on his father's vision, implemented this rigorous testing, establishing a data-driven approach to cable fabrication previously unheard of. This approach, critical for the success of the structure, highlights the shift toward a more scientifically rigorous approach in engineering.
The sheer scale of the Brooklyn Bridge also presented logistical hurdles. Coordinating the workforce, managing material delivery, and ensuring operational efficiency over such a long site – spanning 1,834 feet – presented a formidable management task, especially considering the absence of modern communication technologies. The scale and complexity of the project exposed a critical need for coordination and planning.
Workers within the caissons, in addition to risks associated with "caisson disease", were also faced with shifting sediment and ground conditions that constantly impacted excavation and installation processes. This required rapid adaptations to construction techniques to maintain both progress and worker safety. It demonstrates the necessity of flexibility and creative solutions on a construction site.
Beyond its engineering triumphs, the bridge's construction provided an unexpected window into the past. Artifacts from New York's colonial period were uncovered during the caisson excavations, adding an unexpected layer of historical significance to the project. The bridge's story, therefore, became intertwined with the past, an unexpected consequence of the construction process. It shows the interconnected nature of human endeavors over time.
The bridge's design required a level of precision seldom seen in engineering at the time. The cables, the heart of the structure, needed to be precisely aligned and tensioned to achieve both structural integrity and balance. This degree of precision, focusing on seemingly minor details, played a significant role in ensuring the overall success of the project. This shows a trend towards greater refinement in engineering practices.
Emily Warren Roebling's role as manager of the project after her husband’s illness brought a unique perspective to the forefront. She broke gender barriers while simultaneously showcasing the profound importance of communication and leadership within the field of engineering. It highlights how softer skills can be as crucial as technical knowledge when overcoming obstacles.
Finally, the engineers confronted the challenges of thermal expansion within the bridge's steel components. Temperature variations can cause steel to expand and contract, and these effects were carefully calculated and incorporated into the bridge's design. This foresight was a critical component in ensuring the bridge's long-term stability and demonstrates the evolving sophistication of engineering knowledge and methodologies.
The Roebling Legacy Engineering Marvels Behind the Brooklyn Bridge's Construction - Engineering Techniques That Made It the World's Longest Bridge in 1883
The Brooklyn Bridge's completion in 1883 marked a pivotal moment in engineering, establishing itself as the world's longest suspension bridge at the time. Central to its construction was the pioneering use of steel wire cables, a technique developed by John Augustus Roebling. This innovative approach not only enhanced the bridge's tensile strength but also granted it a crucial flexibility, allowing it to withstand the powerful forces of wind and traffic. The bridge's construction also showcased other advanced techniques. The towering structures, the utilization of pneumatic caissons to create underwater foundations, and the meticulous testing processes all set new standards for future bridge projects. A crucial aspect of Roebling's approach was his emphasis on comprehensive testing and rigorous safety protocols, ensuring the bridge's enduring strength. Ultimately, the success of the Brooklyn Bridge embodies a brilliant fusion of cutting-edge engineering techniques, advanced materials, and a truly visionary engineering philosophy that profoundly impacted the landscape of civil engineering. It stands as a testament to the achievements possible when innovation is combined with a methodical and rigorous approach.
The Brooklyn Bridge, completed in 1883, exemplifies the innovative engineering approaches of the late 19th century. This structure, spanning 5,989 feet across the East River, was the longest suspension bridge in the world at the time, showcasing the ingenuity of John and Washington Roebling's designs. One of the key innovations was the utilization of steel wire cables, a departure from traditional iron structures. This not only increased tensile strength but also remarkably reduced the bridge's overall weight, a crucial aspect for suspension bridges seeking to achieve longer spans without sacrificing stability. The bridge was also designed with a heightened focus on managing dynamic loads, which were those generated by wind and traffic. This was achieved through the composite nature of the cables, a novel approach that allowed them to flex and adapt to these changing forces, a concept significantly ahead of its time in bridge construction.
Another remarkable aspect of the Brooklyn Bridge was the utilization of pneumatic caissons for the underwater foundations. These pressurized chambers, a marvel of engineering for the time, allowed for the excavation and laying of foundations in the riverbed while maintaining safety despite the intense pressure at depths that could reach five atmospheres. However, this technique also introduced previously unknown risks like "caisson disease" for the workers. It was fascinating that despite this danger, it permitted construction to proceed continuously, unhindered by the tides. It is a good example of risk-reward tradeoffs made in design and construction.
The Roeblings' commitment to testing and validating their innovative designs is worth highlighting. Washington Roebling, in particular, oversaw a rigorous testing regimen for the wire cables, measuring their strength and flexibility before their implementation. This scientific approach not only provided vital data for the bridge's design but also helped dispel any initial skepticism about the efficacy of the wire-rope design. It's interesting to reflect on how this marked a gradual but notable shift towards a more data-driven and analytical approach in engineering practice.
Unexpectedly, the construction unearthed a trove of artifacts from early New York settlements, illustrating how human endeavors, even those at the cutting edge of innovation, can provide valuable insights into the past. This intertwined layer of historical discovery, often found serendipitously in large construction projects, reminds us of the ever-present connection to history even as new technologies and structures are built.
The design was also informed by the team's awareness of the thermal expansion and contraction of the steel components. They carefully incorporated calculations that anticipated these temperature fluctuations, demonstrating a level of engineering sophistication seldom seen in that era. Finally, it is important to highlight John Roebling's inclination towards over-engineering, evident in safety factors significantly exceeding two. While this cautious approach was not universally embraced at the time, it has since become an integral part of modern civil engineering practices, cementing the legacy of the Roeblings' vision and their impact on engineering as a field. The Brooklyn Bridge serves as an enduring reminder that a combination of innovation and a meticulous approach to safety can result in structures that not only stand the test of time but also shape the future of engineering.
More Posts from aistructuralreview.com: