Revolutionize structural engineering with AI-powered analysis and design. Transform blueprints into intelligent solutions in minutes. (Get started for free)
Seismic Upgrades and Structural Innovations The Evolution of San Francisco's Bay Bridge Since 1989
Seismic Upgrades and Structural Innovations The Evolution of San Francisco's Bay Bridge Since 1989 - From Devastation to Innovation The 1989 Loma Prieta Earthquake's Impact
The 1989 Loma Prieta earthquake, a magnitude 6.9 event that struck near Santa Cruz, served as a stark reminder of the ever-present seismic risk in the San Francisco Bay Area. This earthquake shattered decades of relative seismic calm, exposing vulnerabilities in the region's infrastructure and causing substantial damage and economic disruption. The event sparked a renewed focus on earthquake preparedness, prompting a wave of seismic research and engineering innovation. This shift resulted in the implementation of advanced structural upgrades, particularly on critical infrastructure like the Bay Bridge, aimed at improving resilience and minimizing the impact of future earthquakes. The lessons learned from Loma Prieta underscore the importance of continuous preparedness and adaptation to ensure the Bay Area's safety in the face of inevitable future seismic events.
The Loma Prieta earthquake, which struck on October 17, 1989, was a stark reminder of the seismic realities of the San Francisco Bay Area. Its magnitude of 6.9 generated ground shaking for approximately 15 seconds, enough to cause significant damage and loss of life. The earthquake's impact was far-reaching, prompting a reevaluation of existing safety standards, especially in the wake of the catastrophic collapse of the Cypress Freeway in Oakland.
The disaster triggered a surge in research into the behavior of bridges and buildings under seismic stress. This led to the development of innovative engineering solutions such as seismic isolation bearings, a massive 1,500-ton seismic gap on the Bay Bridge, and new materials like fiber-reinforced polymers used to strengthen vulnerable structures.
While the Loma Prieta earthquake undoubtedly brought about tragedy, it also spurred a remarkable wave of innovation and a fundamental shift in public perception. This disaster, with its tangible consequences, forced a national conversation about seismic safety and the crucial role of preparedness. This renewed focus resulted in the development of "Caltrans Seismic Design Criteria", which served as a guide for future infrastructure projects across California.
The earthquake also highlighted the need for effective communication and response protocols during disasters. It pushed for increased investment in earthquake early-warning systems, aiming to provide vital seconds of notice before seismic waves hit.
Ultimately, the Loma Prieta earthquake proved to be a powerful catalyst for change, serving as a stark reminder of the need for continuous innovation and vigilance in the face of seismic threats. It pushed the Bay Area, and indeed the entire state of California, to confront its vulnerability and invest in solutions to protect its future.
Seismic Upgrades and Structural Innovations The Evolution of San Francisco's Bay Bridge Since 1989 - Seismic Retrofit Program Launched to Withstand 0 Magnitude Quakes
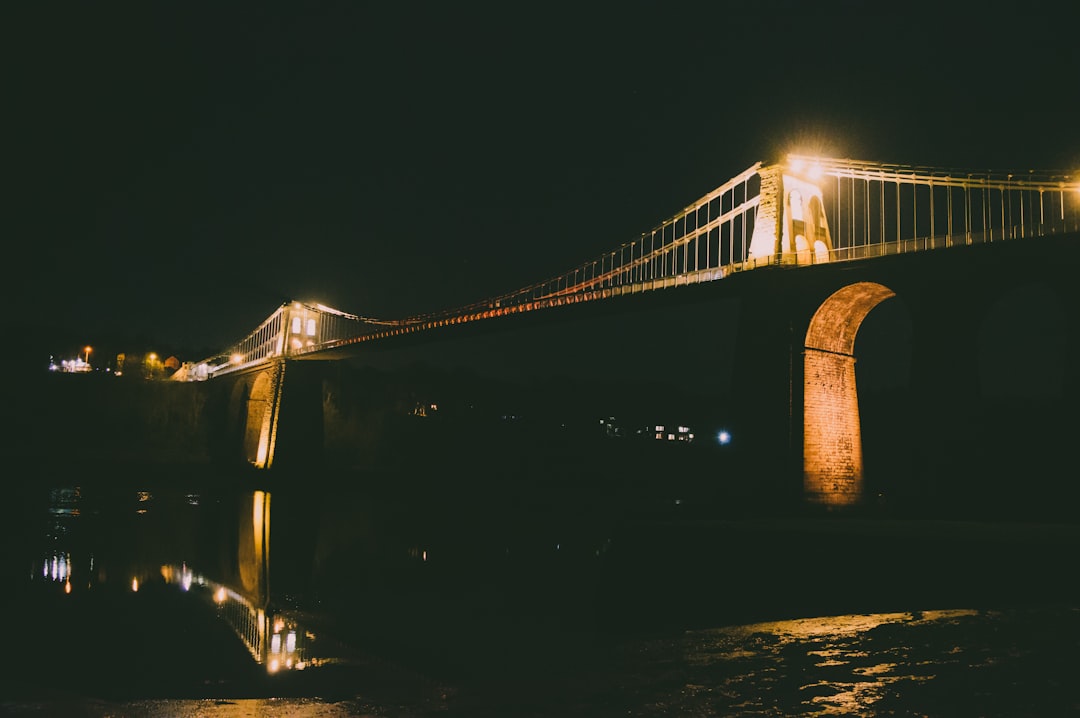
San Francisco has taken a step towards mitigating seismic risk by launching a new seismic retrofit program. This initiative specifically addresses a common vulnerability within the city: wood-framed multifamily soft-story buildings. The average cost for retrofitting these structures is substantial, exceeding $100,000, but it reflects the city's commitment to safeguarding residents and minimizing damage from earthquakes. This program seeks to not only improve safety during seismic events, but also enhance the chances of maintaining habitability in the aftermath. While this effort is a step in the right direction, the city continues to grapple with the ever-present seismic risk and must continually reassess and adapt its strategies in response to the evolving threat.
It's fascinating to see the emphasis on seismic retrofitting, especially for minimal magnitude quakes. While a 0 magnitude quake might seem inconsequential, it highlights the city's commitment to building resilience across the board.
This program goes beyond just structural reinforcement. They are integrating advanced technologies like seismic isolation systems, which effectively decouple structures from the ground motion. It's like building on a platform that can sway independently, minimizing the impact of the quake. This makes me wonder about the practical applications – are we witnessing a shift towards more proactive earthquake preparedness, even for minor events?
The focus on high-performance materials is also interesting. The use of advanced composites and high-strength steel are common in bridges, but the emphasis on ductility is intriguing. It's essentially making the structures more flexible to absorb shock. This is vital to prevent catastrophic failures, especially in structures like the Bay Bridge, which is a vital artery for the region.
The idea of improving seismic performance by as much as 200% is impressive. It raises questions about how these advancements could be applied to existing infrastructure and how the cost-benefit analysis plays out.
Monitoring the stress and displacement data in real-time is a testament to how much we've learned from past events. It allows for continuous feedback and fine-tuning of engineering practices, hopefully, leading to even more resilient structures.
While the program addresses existing structures, the potential economic benefits are also important to consider. The devastating impact of the Loma Prieta earthquake on the Bay Area is a reminder of how much is at stake. Minimizing the economic disruption from even small earthquakes is a significant objective.
Seeing the retrofitting techniques implemented across various architectural styles and materials demonstrates the versatility of modern engineering. It raises questions about how these techniques could be scaled to other regions facing similar seismic risks.
The introduction of energy dissipating devices, like dampers, is a crucial step in protecting the structures from the energy generated during earthquakes. It seems like we're taking a more nuanced approach to seismic mitigation – not just reinforcing, but also actively absorbing the energy.
It's encouraging to see the program being positioned as a model for other regions. It's a reminder that earthquake preparedness is a collaborative effort requiring a combined effort of engineers, policymakers, and communities. This is essential for creating a more resilient future in the face of seismic threats.
Ultimately, learning from past mistakes is paramount. The focus on historical data and case studies is a positive step towards ensuring that future infrastructure developments are more robust and safer.
Seismic Upgrades and Structural Innovations The Evolution of San Francisco's Bay Bridge Since 1989 - West Crossing Upgrades Reinforcing Caisson and Cast-in-Place Foundations
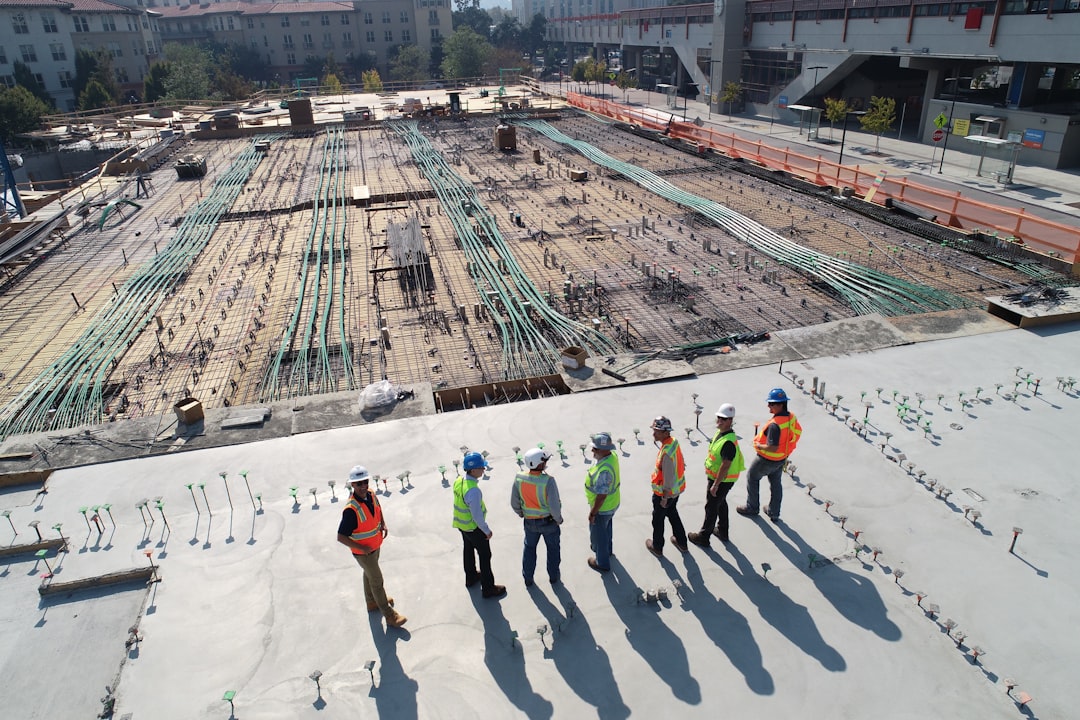
The West Crossing upgrades on the San Francisco-Oakland Bay Bridge involve substantial improvements to its caisson and cast-in-place foundations. This is crucial, given the potential for significant seismic events. The goal is to ensure the bridge remains operational even after a magnitude 8.0 earthquake strikes. To achieve this, the upgrades require careful engineering analysis that models the seismic behavior of the bridge's complex structures. This project also takes into account the impact of the surrounding soil conditions on foundation design, a vital element in creating both strong and safe foundations for the bridge.
These upgrades highlight a larger trend of engineering innovations focused on building resilience against natural disasters, a shift that was especially evident after the 1989 Loma Prieta earthquake. While these efforts are important, the ever-changing nature of seismic risks means that continuous evaluation and adaptation of these upgrades will be essential.
The West Crossing of the San Francisco-Oakland Bay Bridge (SFOBB) presents a fascinating case study in seismic retrofitting. It features six major foundations, a combination of four caisson and two cast-in-place designs. It's captivating how engineers have reimagined these foundations for increased resilience, a critical concern given the constant threat of seismic activity in the region. The caissons, those massive, hollow underwater structures, have been the subject of significant structural enhancements. These upgrades aren't just cosmetic; they involve strategic reinforcement designed to withstand the immense forces unleashed by earthquakes.
It's interesting to note the use of high-performance concrete mixes in the cast-in-place foundations. These mixes are touted for their lower porosity and improved mechanical properties, a significant upgrade that promises extended lifespan and enhanced resistance to seismic stress. Additionally, the application of fiber-reinforced polymers, a lightweight but remarkably strong material, to the exterior of existing foundations is a testament to ongoing engineering innovations. This approach strengthens the structures without imposing additional weight, which could negatively affect the bridge's dynamics during seismic events.
To gauge the effectiveness of these new foundations, real-time monitoring systems have been strategically deployed. This continuous data collection, capturing information like stress, displacement, and vibrations, allows engineers to rigorously assess the bridge's response to seismic activity and make adjustments as needed. This data-driven approach is crucial for fine-tuning the design and ensuring the bridge's continued safety.
The West Crossing project highlights the intricate challenges faced when working with structures of such depth, especially when dealing with caissons that extend 40 feet below the waterline. Such significant depth requires sophisticated excavation techniques to ensure stability and effective reinforcement. I find it fascinating how engineers are adapting their strategies to accommodate the complex logistics and challenges of working with these deep foundations.
The cyclical loading tests conducted on the upgraded structures are a testament to the meticulous nature of modern seismic engineering. These tests are essential to replicate the repeated stresses an earthquake inflicts, allowing engineers to understand how the foundations behave under these extreme conditions. This data informs design modifications that further enhance the caisson's performance in real-world seismic events.
The West Crossing's seismic retrofit exemplifies a shift towards performance-based engineering, an analytical approach that provides a holistic understanding of the bridge's behavior under a range of loads. This approach allows engineers to confidently predict the structure's performance, not only under regular traffic loads but also in extreme seismic scenarios. This data-driven approach ensures that the retrofitting is effective and that the bridge remains safe in the face of potential earthquakes.
The integration of historical data from past seismic events into the design process is an example of learning from experience and applying lessons learned to future endeavors. This data-driven approach ensures that the bridge's upgrades comply with current codes but also anticipate future seismic risks based on real-world experiences, leading to a safer and more resilient bridge.
The implementation of seismic isolation devices, which allow sections of the bridge to move independently during an earthquake, is an innovative approach to reducing the transfer of seismic forces to the main structure. This technology significantly enhances overall stability and reduces the risk of structural damage. This proactive approach to mitigation, focusing on minimizing the transfer of seismic forces, shows the evolution of our understanding of seismic engineering.
Seismic Upgrades and Structural Innovations The Evolution of San Francisco's Bay Bridge Since 1989 - New Eastern Span A 6 Kilometer Self-Anchored Suspension Bridge
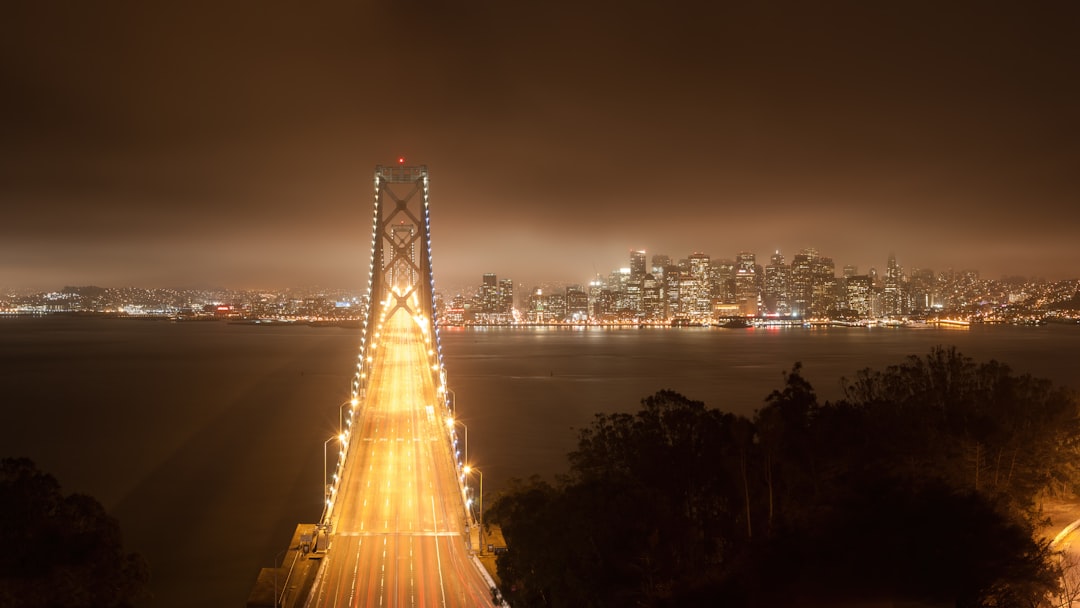
The San Francisco-Oakland Bay Bridge's New Eastern Span, a six-kilometer self-anchored suspension bridge, was completed in 2013. This remarkable feat of engineering stands as a testament to both innovation and resilience in the face of seismic threats. With its 2,047-foot length, the bridge houses the world's longest self-anchored suspension main span. Its construction is a direct response to the vulnerability of past structures exposed by previous earthquakes, demonstrating the ever-present need for seismic safety. The bridge is more than just a sturdy structure; it also includes a multiuse path for pedestrians and cyclists, promoting accessibility and connectivity throughout the region. The New Eastern Span was not without its challenges, experiencing delays and cost overruns that are often part of such ambitious construction projects. Despite these obstacles, its completion exemplifies the ongoing evolution of seismic design and engineering, underscoring the importance of proactively building resilience into urban infrastructure.
The New Eastern Span, spanning 6 kilometers, is an innovative self-anchored suspension bridge with a design unlike any other. Its single, continuous cable running through the deck, eliminating traditional anchorages, reduces the bridge's complexity and footprint. This design makes it one of the world's longest self-anchored suspension spans, stretching a remarkable 1,280 feet. This accomplishment isn't without challenges, as engineers had to contend with the immense weight and dynamic forces, like wind and seismic activity, when creating it.
This bridge is engineered to withstand seismic events as powerful as magnitude 8.5, showcasing a crucial shift towards a "progressive collapse" design. This design means that even if a localized failure occurs, the entire structure won't collapse, emphasizing resilience and survival. Its distinctive "Y" shape provides optimal stress distribution across the span, showcasing sophisticated structural mechanics and a clear understanding of vertical and lateral loads. This configuration contributes significantly to the bridge's overall stability.
Modular construction techniques played a vital role in the bridge's development. By pre-fabricating and assembling various sections on-site, the construction process was expedited while also improving quality control and ensuring structural integrity. The incorporation of advanced energy-dissipation devices is a critical feature for withstanding the dynamic forces experienced during earthquakes. These dampers effectively absorb and dissipate the energy generated by ground motion, minimizing potential structural damage.
The New Eastern Span boasts an innovative sway brace system designed to enhance lateral stability. This system effectively counters the swaying motion that occurs during earthquakes, ensuring the bridge remains stable under dynamic loads. The deck's placement significantly higher than its predecessor increases clearance for marine traffic, allowing larger vessels to pass freely without compromising the bridge's resilience.
The incorporation of fiber-reinforced composites in critical components contributes to the bridge's durability while maintaining a lighter weight. This material minimizes stress on the supporting structures, a crucial element during seismic events. Real-time monitoring systems have been integrated throughout the bridge, providing continuous assessments of its performance. This approach allows engineers to identify any signs of distress proactively, reinforcing the focus on long-term sustainability in bridge engineering.
Seismic Upgrades and Structural Innovations The Evolution of San Francisco's Bay Bridge Since 1989 - Single Tower Design Chosen by International Expert Panel
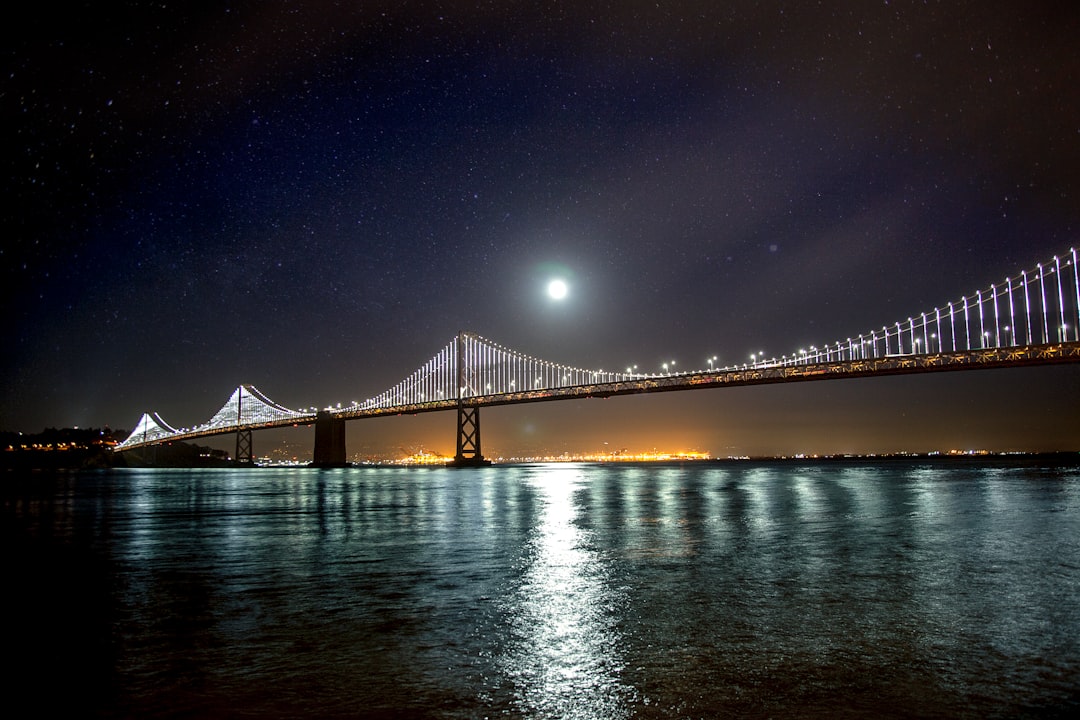
The San Francisco-Oakland Bay Bridge has taken a significant step toward enhancing its resilience against earthquakes. An international panel of experts has chosen a single-tower design for the bridge's replacement structure. This design prioritizes structural integrity and seismic safety, making it a cornerstone of modern seismic engineering.
The single tower design is not only intended to support the weight of the bridge but also to counter lateral forces that could occur during earthquakes. The tower's mass and stiffness characteristics act as a buffer against these forces, ensuring the bridge's stability during seismic events.
This decision reflects a commitment to innovative approaches in structural design, showcasing the dedication to creating resilient infrastructure. This design is a testament to the ongoing evolution of engineering principles and their application in protecting urban infrastructure from the ever-present threat of earthquakes. The single-tower design is a crucial step in bolstering the Bay Bridge's ability to withstand the rigors of seismic activity, ensuring its safety and functionality for the years to come.
The Bay Bridge's New Eastern Span is a testament to the evolution of seismic engineering since the 1989 Loma Prieta earthquake. The decision to utilize a single tower design for the bridge was made after careful consideration by an international panel of experts. This choice, while seemingly simple, carries significant implications. By eliminating the need for multiple towers, the design simplifies construction, minimizes the bridge's overall footprint, and potentially reduces costs. However, it also highlights the reliance on innovative engineering principles to maintain structural integrity.
The engineers opted for a self-anchored suspension bridge design, a significant departure from traditional structures. This design involves a continuous cable system that eliminates the need for expansive anchorages, which allows for a more streamlined and efficient construction process. The bridge is also designed to endure seismic forces up to an 8.5 magnitude earthquake, a testament to the proactive shift towards building resilience in the face of potential natural disasters. This emphasis on robust structural performance is evident in the bridge's unique "Y" shape. This configuration not only provides a distinctive aesthetic but also optimizes the distribution of stress, ensuring the structure can withstand the powerful forces associated with earthquakes.
Modular construction techniques, used during the building process, added to the bridge's success. Pre-fabricating and assembling sections on-site accelerated construction, minimizing potential delays and enhancing quality control. This approach not only improved efficiency but also reduced waste, ultimately contributing to a safer and more sustainable construction project. The bridge is also equipped with real-time monitoring systems that provide engineers with continuous data regarding its structural integrity. This allows them to identify any potential issues quickly, reinforcing the focus on long-term safety and maintenance.
The New Eastern Span incorporates a series of advanced energy dissipation devices that serve as shock absorbers during seismic activity. These dampers effectively mitigate the impact of ground motion, minimizing the risk of catastrophic structural failure. Furthermore, the use of fiber-reinforced composites, known for their durability and lightweight nature, enhances the bridge's longevity. These materials offer superior resistance to environmental degradation compared to traditional materials, making the bridge more resilient over time.
The New Eastern Span also features a multi-use path for pedestrians and cyclists, highlighting a new approach to designing infrastructure. This demonstrates that advancements in structural engineering can seamlessly integrate with urban design, improving accessibility and connectivity for all users. With its 2,047-foot main span, the bridge holds the title of the world's longest self-anchored suspension span, a testament to the continued advancement of engineering in the pursuit of innovation and efficiency. This innovative structure exemplifies the dynamic interplay of science and engineering, and serves as a powerful reminder of the importance of continuous adaptation in the face of evolving challenges.
Seismic Upgrades and Structural Innovations The Evolution of San Francisco's Bay Bridge Since 1989 - Instrumentation for Real-Time Seismic Performance Analysis
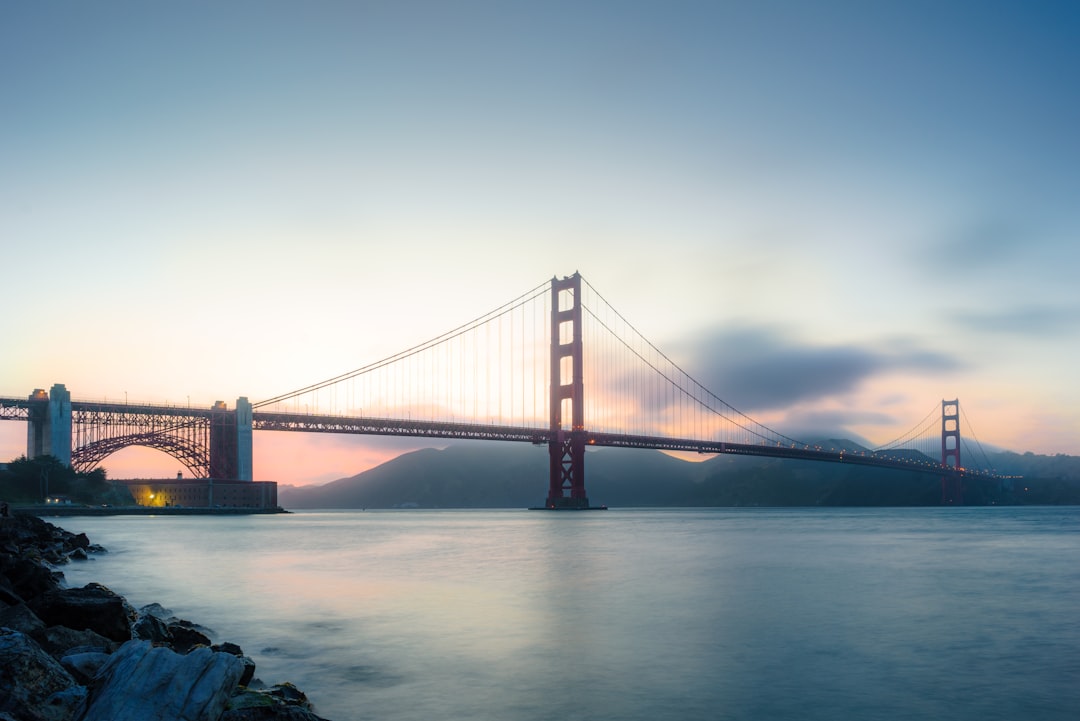
The San Francisco Bay Bridge, a testament to engineering resilience after the 1989 Loma Prieta earthquake, has incorporated a new layer of safety: real-time seismic performance analysis. This involves sophisticated systems that constantly monitor the bridge's response to seismic activity. Imagine having a detailed, real-time picture of how the bridge is reacting to the ground shaking during an earthquake! This is achieved through advanced techniques like Proper Orthogonal Decomposition (POD) and Proper Generalized Decomposition (PGD), which allow engineers to analyze structural behavior with incredible detail. These insights are crucial for making informed decisions during an earthquake, perhaps even triggering adjustments to the bridge's systems in real-time to minimize damage.
This isn't a new idea, but the technology is constantly evolving. Networks like the Southern California Seismic Network, with hundreds of seismometers, provide unprecedented data on ground motion. This means we're getting a more accurate picture of how earthquakes actually affect buildings. The integration of these data-driven insights into real-time monitoring systems allows engineers to refine their understanding of how structures perform under seismic stress, essentially allowing them to learn from each earthquake. It's like having a virtual earthquake simulator for the Bay Bridge, constantly providing feedback on its resilience. This commitment to continuous learning is crucial for a region that's inherently vulnerable to seismic events.
The Bay Bridge's seismic upgrades are a fascinating example of how engineering has evolved since the 1989 Loma Prieta earthquake. The focus now is on real-time monitoring and data-driven decision-making. For instance, the bridge is equipped with advanced sensors that track things like acceleration, strain, and displacement during seismic events. This gives engineers vital information about the bridge's health during an earthquake.
Another interesting development is the use of seismic isolation systems. These essentially decouple the foundation from the superstructure, reducing the transfer of seismic forces and giving the bridge more flexibility during an earthquake. This is a great example of how engineers are shifting their focus from just strengthening structures to actively mitigating the impact of earthquakes.
Energy dissipating devices, like viscous dampers, are also becoming more common. These act like shock absorbers and are key for preventing catastrophic failures in structures. The dampers absorb the energy released during seismic events, minimizing the stress on the bridge and increasing its resilience.
The modern approach to seismic design is also rooted in historical data. Engineers use complex computational models to simulate past earthquakes, helping them better understand how a structure might behave in a future event. This data helps inform the design and allows for a more comprehensive understanding of potential vulnerabilities.
Fiber-reinforced composites are increasingly used in upgrades because they offer a combination of high strength and low weight. This means they can strengthen existing structures without adding significant weight, which is important for maintaining the dynamic balance of the bridge during earthquakes.
The overall philosophy behind these modern upgrades is performance-based design, which focuses on how a structure will actually perform under different loads, including seismic events. This approach ensures that the bridge is not only compliant with current codes but also resilient to future seismic threats.
There's even more to come in terms of integrating machine learning into the monitoring system. The idea is to identify patterns and anomalies in real-time data, which could help predict potential structural failures. This is a promising new development that has the potential to revolutionize our approach to seismic risk management.
But while these advancements are impressive, there are still challenges. Sensors need to be reliable in harsh environments, and calibration and maintenance are critical for ensuring accurate data collection. We need to keep in mind that inaccurate data could lead to flawed assessments and potentially dangerous decisions.
It's exciting to see how engineering is adapting to the challenges of seismic risk. The ongoing research and development, combined with the implementation of innovative technologies, are paving the way for a more resilient future.
Revolutionize structural engineering with AI-powered analysis and design. Transform blueprints into intelligent solutions in minutes. (Get started for free)
More Posts from aistructuralreview.com: