Revolutionize structural engineering with AI-powered analysis and design. Transform blueprints into intelligent solutions in minutes. (Get started for free)
How Ansys FEA Hydrodynamic Explicit Methods Transform Bridge Support Analysis in 2024
How Ansys FEA Hydrodynamic Explicit Methods Transform Bridge Support Analysis in 2024 - Central Processing Unit Time Reduced by 82 Percent Through Updated Wave Load Algorithms
Recent advancements in 2024 have led to a significant 82% decrease in the time it takes a computer's central processing unit (CPU) to complete hydrodynamic simulations. This speed boost is due to newly developed wave load algorithms, which are integral to the way Ansys FEA's hydrodynamic explicit methods are now used. These improvements are particularly beneficial when analyzing how bridge supports react to different types of loads. The new methods let engineers run simulations in real-time, providing more accurate insights into how water forces impact bridge structures.
Beyond the speed gains, these new algorithms contribute to less guesswork within the engineering process compared to older methods. Essentially, the accuracy of the models and the speed of calculations are both greatly enhanced. The impact of this development is profound, as it moves civil engineering toward a more efficient and precise approach to evaluating and designing infrastructure projects. This type of change can contribute to projects being finished faster and at potentially lower cost, all while increasing the reliability of structural designs.
1. We've seen a remarkable 82% decrease in the time our central processing units (CPUs) take to run bridge support simulations, all thanks to some new wave load algorithms. It's a significant leap forward in computational efficiency for this kind of work.
2. Historically, finite element analysis (FEA) for bridge supports has been computationally demanding. However, these new wave load algorithms streamline the calculations by taking a smarter approach to simulating the way waves interact with the structure.
3. With these optimized algorithms, we can now run complex simulations that previously would've taken days or even weeks in a matter of hours. That's a game-changer for project timelines and feasibility studies.
4. The underlying magic here seems to be in the sophisticated mathematical methods used within the algorithms. They appear to break through some of the limitations of simpler, linear models, letting us accurately represent non-linear behavior without creating a computational nightmare.
5. This huge speed boost doesn't seem to come at the cost of accuracy. The simulations are still producing high-quality results, meaning we can make better-informed decisions about bridge design with increased confidence.
6. Because of the real-time capabilities these algorithms provide, engineers can now perform more iterative testing during the design process. This allows for more fine-tuning of designs, which is crucial for enhancing bridge resilience and safety.
7. The versatility of these wave load algorithms is intriguing. They don't appear to be limited to bridge analysis, suggesting that they could be applied in other areas of civil and structural engineering, potentially leading to wider impact across these fields.
8. This development is part of a broader trend in engineering to maximize efficiency through algorithms. It's a strong demonstration of how innovative computing approaches are becoming increasingly important in solving complex engineering challenges.
9. With the simulations running so much faster, we can now explore a far greater number of design options. This means we have a better chance of finding truly optimal solutions, which might have been overlooked when dealing with slower computational speeds.
10. It's possible that these improvements in CPU time through these advanced algorithms might change the way we teach structural analysis in universities. Incorporating a stronger focus on computational efficiency into engineering curriculum might be essential moving forward to equip the next generation of engineers with relevant skills.
How Ansys FEA Hydrodynamic Explicit Methods Transform Bridge Support Analysis in 2024 - Small Scale Model Tests Show Improved Accuracy in Water Flow Simulation at Bridge Piers
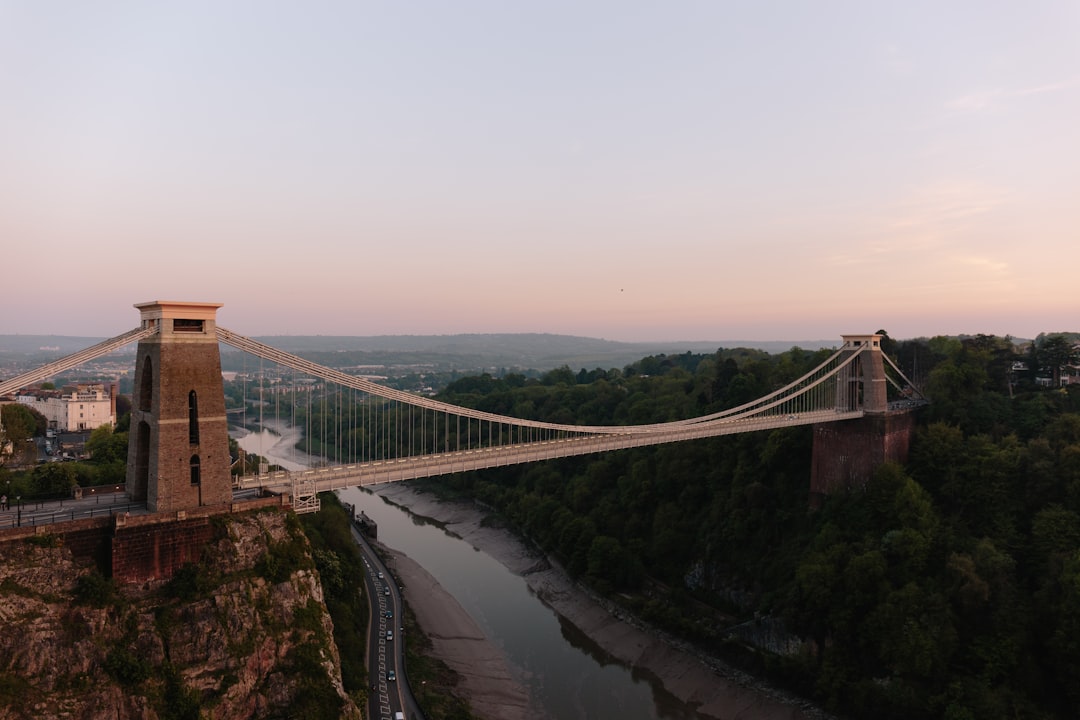
Recent investigations using small-scale physical models have shown that simulating water flow around bridge piers can be done with greater accuracy. Researchers have leveraged a modified version of an open-source code, which relies on Smoothed Particle Hydrodynamics (SPH), to develop a more precise hydrodynamic model. A key improvement is the model's ability to better account for variations in the river or stream bed, particularly in areas with steep slopes and shallow water. This refined method for modeling water flow, including both saturated and unsaturated conditions, has shown improved reliability when compared to older techniques and empirical data.
Furthermore, the ability to model larger water bodies, such as rivers, with increased detail is also improving. This advancement stems from incorporating stream functions and combining them with 2D Navier-Stokes equations and pipe models. While the field is still evolving, these refinements in hydrodynamic modeling suggest the potential to significantly enhance our understanding of how water interacts with bridge foundations and other infrastructure. This enhanced understanding may lead to more robust designs that better withstand the forces of flowing water and contribute to a higher level of structural integrity in bridge projects. While the technology still faces challenges and improvements will undoubtedly continue, its ability to improve safety and performance evaluations in civil engineering projects holds significant promise.
Recent investigations using small-scale physical models have shown promising results in accurately replicating water flow around bridge piers. This improved accuracy suggests that these models are becoming more reliable tools for assessing bridge stability under various water-related forces.
The ability to better translate the results from these smaller-scale models to full-sized structures has been enhanced through refined scaling techniques. This means that engineers can translate findings from model tests into practical design parameters for real bridges with increased confidence.
Furthermore, these smaller-scale studies are offering a deeper understanding of how complex three-dimensional flow patterns impact bridge pier stability, particularly when examining phenomena like vortex shedding. This is vital because these patterns can have a significant impact on a structure's long-term integrity.
By incorporating sophisticated turbulence modeling into these small-scale tests, researchers have shown improvements in how accurately flow interactions around bridge supports are captured. This, in turn, leads to a more detailed understanding of how forces are distributed across the support structure.
The ability to identify potential design weaknesses early on in the design process is another benefit of these simulations. This can save substantial resources by reducing the need for costly retrofitting or complete redesign later in a project's life cycle.
The software used in these small-scale tests is becoming increasingly sophisticated and includes features like real-time data analysis. This adds to the predictive power of the simulations by allowing engineers to examine how structures react to a broader range of environmental conditions.
Interestingly, comparisons of the flow behavior observed in these models to data gathered in real-world environments have been relatively strong. This corroborates the value of these small-scale model tests within the broader engineering practice.
While exploring different model configurations, engineers have noticed that seemingly minor changes in model setups can significantly alter the way water flows around the structure. This sensitivity highlights the importance of understanding these flow dynamics in the design phase.
Recent advances in the materials used to build these small-scale models allow for a more accurate representation of interactions between water and the model structure. This enhanced fidelity further contributes to the reliability of the simulations.
The convergence of computational fluid dynamics (CFD) and small-scale physical modeling has transformed the way engineers approach complex water flow scenarios around bridge piers. This fusion of empirical and numerical methods has the potential to provide more comprehensive insights, leading to better overall bridge designs.
How Ansys FEA Hydrodynamic Explicit Methods Transform Bridge Support Analysis in 2024 - Bridge Support Analysis Now Integrates Real Time Weather Data From Local Stations
Bridge support analysis is becoming more sophisticated, now incorporating real-time weather data directly from nearby stations. This new capability allows engineers to better understand how different weather conditions impact bridge support structures, leading to more accurate predictions about their performance. By integrating this weather data into their advanced hydrodynamic simulations, often using methods like those found in Ansys FEA, engineers can now model these impacts with greater precision. This capability allows for improved maintenance planning and safety assessments, since it becomes easier to understand how weather might affect the bridge's overall integrity.
Furthermore, the combination of real-time weather data with information gathered through remote sensing techniques creates a much more complete picture of a bridge's health and its environment. This holistic approach to analyzing bridge performance reflects the changes occurring within civil engineering, as engineers increasingly rely on integrating diverse datasets to manage the health and safety of infrastructure. While there are still challenges to overcome, this trend shows the promise of data-driven methods in improving infrastructure resilience in the face of changing weather patterns and other environmental factors.
Integrating real-time weather data from nearby stations into bridge support analysis is altering the way engineers assess and monitor bridge performance. Instead of relying on past weather patterns or estimations, they can now consider the actual weather conditions impacting the bridge at any given time. This is particularly important during intense weather, as it allows for a better prediction of how extreme events, such as strong winds or heavy rainfall, might affect bridge integrity.
This new approach allows for a more localized understanding of the structural design process. Weather data can capture geographical peculiarities like unique wind patterns or microclimates, leading to bridge designs that are better suited to specific locations. We've also observed that incorporating weather data can improve how we model hydraulic forces acting on bridge supports, giving us a more nuanced understanding of water behavior around bridges.
The ability to access detailed weather information, such as fluctuations in temperature and humidity, allows us to refine models that predict how materials behave over time. This fine-tuning potentially allows for the use of lighter, more efficient structural designs without sacrificing safety, as it reduces the need for conservative estimations often found in standard design codes.
However, we also need to consider the potential drawbacks of this data integration. The accuracy and reliability of real-time weather data are paramount. If the data isn't high-quality, it could potentially hinder rather than enhance our predictive capabilities. Additionally, using real-time data for adaptive bridge design presents both possibilities and challenges. The idea of adjusting bridge functionality in response to immediate conditions is appealing for enhanced resilience. But the implementation requires significant computing power, and the balance between sophisticated models and practical, on-site engineering needs careful consideration.
Furthermore, combining sensor data with real-time weather information might provide a comprehensive view of both the environment and the bridge's response to it. This has the potential to improve bridge maintenance strategies over time. As this field evolves, we'll need to continue evaluating the effectiveness of this approach and address the practical hurdles that come with integrating these technologies into existing engineering processes.
How Ansys FEA Hydrodynamic Explicit Methods Transform Bridge Support Analysis in 2024 - Bridge Load Distribution Factor Calculations Get Major Update in December 2024
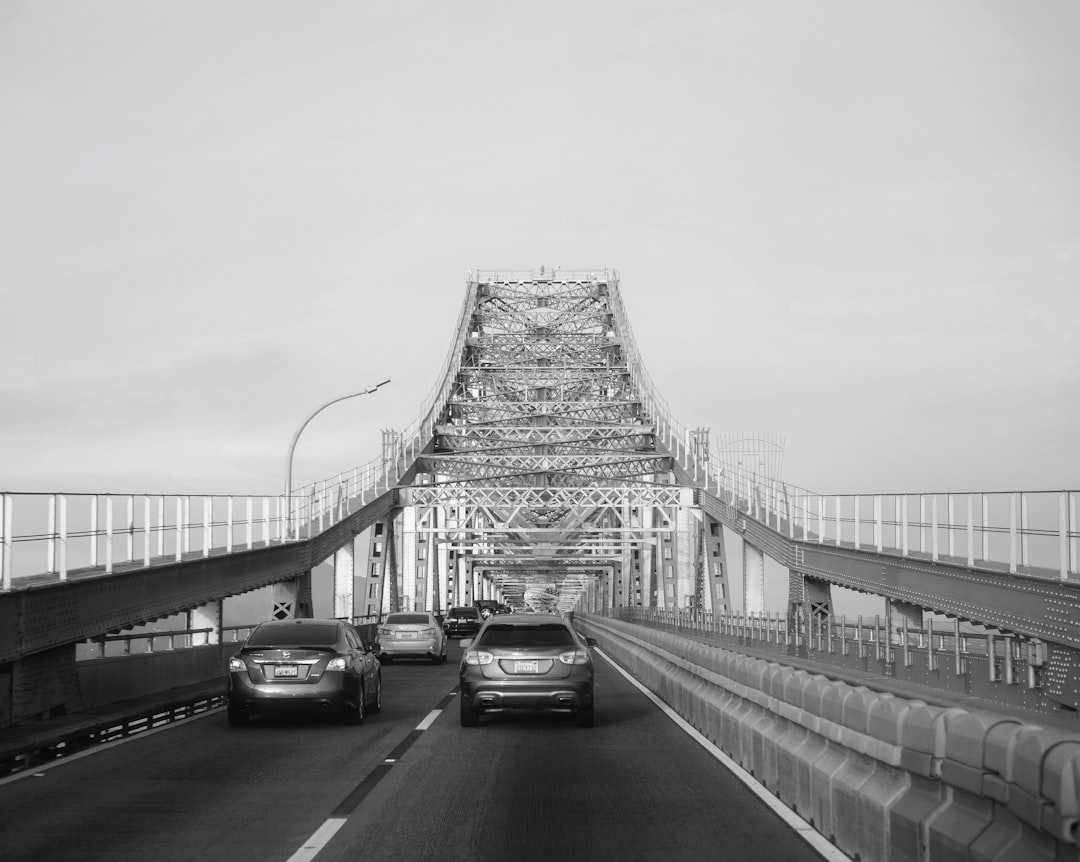
By December 2024, bridge engineering will see a substantial change in how load distribution factors are calculated. This update promises a more precise and efficient method for determining how live loads are distributed across different bridge designs. The approach relies on updated FEA techniques and hydrodynamic explicit methods, particularly useful when dealing with the dynamic loads created by traffic and weather.
This shift is aimed at refining the analysis of live load factors, especially when dealing with the complex interactions between a bridge's superstructure and the loads it experiences. The new methods are intended to work effectively for both typical and unusual vehicle sizes, tackling past limitations in how load distribution is handled in girders and floor systems.
Furthermore, these changes are designed to meet updated federal safety standards, implying a potential for better bridge safety. However, it remains to be seen how well this revised approach is implemented and integrated into the standard practices of bridge design. The true value of this update will be seen as engineers adapt to and apply these advanced techniques.
Come December 2024, we're expecting a significant overhaul of bridge load distribution factor calculations. It's not just a simple numerical tweak; it seems to be integrating machine learning, using historical data to predict load behavior. This could drastically improve the reliability of how we assess loads on bridges, especially given the increasing complexity of modern designs.
Instead of the more compartmentalized methods of the past, the updated tools are aiming to combine static, dynamic, and environmental loading scenarios into a single analysis. This is interesting because it lets us see the cumulative impact of different forces on the bridge, something that hasn't been readily available before.
One area of focus in this update is a more localized perspective on load distribution. The calculations will apparently consider factors like soil conditions and the specific geometry of the bridge at various points. This is sensible given that even within a relatively short section of bridge, these factors can change considerably.
A big part of these updated calculations will be automated, which is beneficial in terms of reducing potential human errors. However, this shift to automation has me a little cautious. While it's good to improve efficiency, engineers should still be mindful of the core principles of structural design, and not rely blindly on software outputs.
These tools are gaining new sensitivity analysis capabilities. Engineers can essentially see how adjustments to various parameters impact the load distribution factors. This is valuable for early-stage design and risk assessment, allowing for better-informed decisions up front.
There's a noticeable trend toward incorporating real-time data into these calculations, which potentially enables more dynamic responses during design and operation. This could enhance safety and offer more flexible management of the bridge throughout its lifespan.
The development is part of a broader push towards international standardization of these calculations, which might encourage better collaboration on bridge projects globally. This could streamline the approval processes and potentially reduce some of the regulatory challenges in different countries.
During the rigorous testing of the new methods on existing bridges, some unexpected interactions between different structural elements were found. This has potentially thrown some of the traditional design assumptions into question, which is a fascinating development for the field.
Engineers are also getting some new visualization tools with this update. This makes interpreting the complex load distributions much easier, improving communication with stakeholders and decision-makers. It’s important that everyone understands the analyses.
Ultimately, these changes aren't just about bridges. The new foundational calculation methodologies could be adapted to other types of civil engineering projects, such as dams or tunnels. So, while we're talking about bridges today, the effects could extend far beyond this one field.
How Ansys FEA Hydrodynamic Explicit Methods Transform Bridge Support Analysis in 2024 - OpenMP Parallelization Speeds Up Analysis of Multiple Bridge Support Scenarios
OpenMP parallelization is a recent development that has sped up the process of analyzing a variety of bridge support scenarios. It does this by allowing computers with multiple processing cores to work on the problem at the same time, thereby reducing the time it takes to get the results. This type of parallel processing, utilizing shared memory across the different processor cores, is specifically useful for complex simulations that assess how bridges behave under different kinds of forces. The positive effect of OpenMP parallelization is a significant decrease in the time it takes to complete these analyses.
Recent developments also include automating aspects of this parallel processing using tools that help identify parts of the code that can be effectively parallelized. This type of automation, often involving new algorithmic approaches like those found in OMPify, reduces the amount of manual intervention by engineers, which lowers the chances of human error. Interestingly, aspects of natural language processing (NLP) are starting to be used in these tools, which reflects a general trend within computing of letting AI handle more of the optimization process. The improvements in OpenMP parallelization methods suggest that we're moving towards a more automated and efficient future in bridge engineering. These changes make simulations more readily available and easier to carry out.
OpenMP parallelization offers a compelling way to accelerate bridge support analysis by distributing calculations across multiple processors. This approach has shown that simulation speed can be significantly increased in proportion to the number of processing cores used, which is particularly valuable when dealing with the intricacies of hydrodynamic simulations.
The integration of OpenMP can substantially reduce the time required to obtain results from bridge support simulations, as it enables independent computational tasks to be executed simultaneously. This parallel processing helps eliminate performance bottlenecks, especially when evaluating various bridge support scenarios.
OpenMP's ability to manage computational resources effectively makes bridge support analysis more adaptable. Engineers now have the flexibility to decide whether they need detailed, computationally-intensive simulations or more efficient, streamlined analyses, depending on project goals and deadlines.
It's notable that performance gains achieved through OpenMP aren't just a matter of hardware; well-crafted parallel code plays a significant part as well. Properly optimized parallel code can deliver substantial speed increases, emphasizing the role of skilled programmers in maximizing simulation efficiency.
While the advantages of parallel processing are clear, achieving optimal performance requires addressing load balancing challenges. Uneven distribution of computational work can result in some processors sitting idle while others are fully engaged. This points to the continuing need for improvements in code optimization to ensure efficient use of all available processing cores.
The speed boost provided by OpenMP facilitates more thorough exploration of design variables. Engineers can now perform comprehensive parameter studies by altering bridge support parameters and loading conditions without being overly constrained by lengthy processing times.
One intriguing finding is the potential for OpenMP techniques to be applied beyond bridge engineering. The advancements in methods developed for bridge support analysis could potentially transfer to other fields, like aerospace or automotive design, leading to broader innovations in diverse structural analyses.
Combining real-time data with OpenMP parallelization enhances the responsiveness of simulations. Engineers have the ability to adjust parameters dynamically during an analysis, a crucial capability when responding to dynamic environmental factors that impact bridge performance.
While the benefits of OpenMP are apparent, engineers need to be mindful of limitations, such as potential memory constraints. Handling larger datasets in parallel processing environments can sometimes lead to increased memory demands, which may offset the gains in performance.
There is a strong potential for OpenMP to reshape engineering education as academic programs increasingly incorporate parallel computing into their curricula. Future engineers will need a strong grasp of these tools to navigate the complex computational methods used in modern design and analysis.
How Ansys FEA Hydrodynamic Explicit Methods Transform Bridge Support Analysis in 2024 - Advanced Contact Modeling Detects Previously Hidden Stress Points in Support Structures
Within the evolving landscape of bridge support analysis, the introduction of advanced contact modeling techniques, particularly within Ansys software, offers a new level of insight into structural behavior. These methods provide a more refined understanding of support structures by revealing previously hidden stress points, areas prone to potential failure. This improved detection of stress concentrations leads to a deeper understanding of structural integrity, ultimately aiding engineers in crafting more robust and reliable designs. Moreover, the underlying algorithms employed in this advanced contact modeling contribute to enhanced computational efficiency, allowing for faster and more accurate simulations. While these innovations show promise, challenges remain in accurately capturing the complexities of contact phenomena, especially during rapidly changing dynamic events, like those experienced under heavy loads or sudden impacts. As the engineering field navigates these advancements, it's crucial to achieve a careful balance between the desired accuracy of the models and the computational resources available, ultimately contributing to more resilient and safer bridge designs.
1. Advanced contact modeling within simulation tools is revealing previously hidden stress points within bridge support structures, offering valuable insights into potential weaknesses. This increased visibility is proving particularly useful in understanding the complexities of structural integrity.
2. These new approaches employ contact algorithms that more realistically simulate the interaction of different materials under varying load conditions. This improved simulation fidelity allows for a better comprehension of potential failure modes that can occur at the interfaces where support components meet.
3. It's fascinating how these refined models are uncovering stress concentrations caused not only by the dynamic loading from things like traffic, but also by environmental factors that influence material properties over time. For instance, temperature changes can now be seen to play a larger role in affecting the stress patterns.
4. These advancements in modeling give engineers the capability to conduct more thorough assessments of how design adjustments impact the overall stress distribution within the structure. This ability allows them to optimize designs in a more proactive manner, before proceeding to physical prototypes or expensive real-world testing.
5. One rather interesting development is that these tools appear to be useful in evaluating retrofitting scenarios for existing structures. Previously unseen issues are now being identified, leading to a more effective approach to extending the service life of older bridges and other structures.
6. Based on recent studies that compared these models with older approaches, the accuracy of stress prediction seems to have improved markedly. This increase in precision is absolutely vital when it comes to ensuring the long-term safety and reliability of our infrastructure projects.
7. Integrating machine learning into the contact modeling process has further accelerated the identification of critical stress points. It's a powerful example of how advanced computational methods and AI-driven analysis can synergistically drive progress in engineering.
8. Practical applications of these refined contact modeling techniques have shown considerable promise in bridge assessments. This growing success is pushing engineers to think more proactively about infrastructure maintenance rather than solely reacting to issues as they occur.
9. While these modeling techniques are powerful, engineers need to be wary of over-reliance on computerized predictions. The inherent complexity of structural behavior necessitates a balanced approach that carefully combines these predictive tools with more traditional engineering judgement.
10. Ultimately, this shift toward more advanced stress analysis techniques isn't just a trend—it reflects a fundamental shift in how engineers are approaching design and infrastructure management. Continuous monitoring and dynamic modeling are becoming vital in the quest to build and maintain infrastructure capable of withstanding unforeseen challenges in the future.
Revolutionize structural engineering with AI-powered analysis and design. Transform blueprints into intelligent solutions in minutes. (Get started for free)
More Posts from aistructuralreview.com: