Revolutionize structural engineering with AI-powered analysis and design. Transform blueprints into intelligent solutions in minutes. (Get started for free)
Load Distribution Analysis Why Modern House Foundations Fail Under Lateral Forces
Load Distribution Analysis Why Modern House Foundations Fail Under Lateral Forces - Soil Liquefaction During 2011 Christchurch Earthquake Led To Foundation Failures
The Christchurch earthquake of 2011 starkly illustrated the fragility of conventional house foundations in the face of rapid soil liquefaction. This process, unfolding in a matter of seconds, primarily impacted residential areas and the city's core, triggering widespread structural failures. Lateral spreading and ground deformation became prominent features of the damage, showcasing how many building foundations were unprepared for the intensity of lateral forces generated during the earthquake. The severity of the damage highlighted deficiencies in established foundation design methods, prompting a shift in building regulations and urban planning. A greater emphasis emerged on understanding how liquefaction impacts soil properties and influences future earthquake resilience. This event emphasizes the need for more rigorous analysis of load distribution in foundation design to guarantee stability in extreme situations.
The 2011 Christchurch earthquake sequence, featuring multiple strong events, dramatically illustrated the destructive potential of soil liquefaction. This rapid loss of soil strength, often occurring within seconds, became particularly evident in the city's eastern suburbs. Liquefaction's impact extended beyond isolated incidents, causing widespread damage to residential areas and the city's core.
The earthquake's severity, combined with the specific soil conditions in Christchurch, resulted in substantial ground deformation through lateral spreading. This phenomenon, a direct consequence of liquefaction, inflicted heavy damage on various infrastructure, including residential structures, essential bridges, and critical water supply lines. Buildings, especially those located in the central business district, experienced noticeable deformations as the ground shifted beneath them.
The aftermath revealed that the soil's physical properties were significantly altered by liquefaction, posing a formidable challenge for the stability of modern house foundations when subjected to lateral forces. It became clear that traditional foundation designs were insufficient to withstand the intensity of the lateral forces encountered during the earthquake sequence. These earthquakes, including both shallow and deep events, triggered nonlinear soil behavior under the intense ground shaking.
The Christchurch earthquakes served as a stark reminder of the need to improve the understanding of liquefaction's influence on urban planning and foundation design. This realisation prompted a deeper examination of building codes and design practices. The importance of incorporating soil characteristics and susceptibility to liquefaction into the initial stages of design is now more widely acknowledged, with an emphasis on solutions such as deeper foundations and soil improvement techniques in vulnerable areas. The impact of this earthquake continues to shape global building standards as a valuable case study illustrating the essential role of geological assessments in reducing future risks related to soil liquefaction.
Load Distribution Analysis Why Modern House Foundations Fail Under Lateral Forces - Inadequate Wind Load Distribution Paths Through Modern Open Floor Plans
Modern homes often feature open floor plans, prioritizing spaciousness by minimizing interior walls. However, this architectural trend can inadvertently create challenges for managing wind loads. The absence of numerous internal walls disrupts the traditional pathways for distributing lateral forces, which are typically managed by shear walls. These shear walls, acting as vertical diaphragms, rely on a robust connection to the building's structure to effectively resist wind forces. Without a clear path to transmit these forces, the building's ability to maintain stability during high winds can be compromised.
The geometry of a building and its overall mass are significant factors in how wind loads impact the structure. Open layouts can complicate this relationship, creating an uneven distribution of forces and potentially causing undue stress on certain areas. A deeper understanding of how wind interacts with the building's design is crucial to ensure safety in these increasingly popular home layouts. If not addressed during the design phase, the absence of sufficient wind load distribution paths could contribute to structural issues, particularly in locations prone to high wind events. Ultimately, the widespread adoption of open floor plans highlights the need for reevaluating how architectural design influences the building's structural integrity, especially when it comes to withstanding lateral loads.
Modern open floor plans, with their emphasis on spaciousness and minimal internal walls, often present a challenge for distributing lateral loads effectively. This can lead to structural vulnerabilities, especially during events like strong winds or earthquakes. The absence of traditional load-bearing elements, such as shear walls, can cause the structure to twist or 'torsion' under lateral forces, potentially concentrating stress at weak points and increasing the risk of failure.
High ceilings, a common feature in such designs, can exacerbate the problem. The increased height leads to greater potential for uplift forces on the roof, which creates significant moments at the roof-to-wall connection and potentially amplifies structural vulnerabilities. Furthermore, the trend toward expansive glass facades in these designs further complicates the issue. Glass, while aesthetically pleasing, is not as structurally robust as solid materials in resisting lateral loads. This raises concerns about the structural integrity of façades during extreme weather events.
Current engineering codes, which often rely on traditional building layouts, may not adequately address the unique challenges posed by modern open floor plans. This can lead to a discrepancy between the predicted performance of these structures and their actual behavior under lateral forces. Traditional bracing elements, such as framed structures, that contribute to stability during high-stress events are often reduced or removed in modern open plans, further weakening their lateral load resistance.
Limited spacing between structural elements can also create 'bottlenecks' in the load paths, hindering the structure's ability to distribute forces efficiently. With climate change increasing wind speeds, the risk associated with insufficient load distribution becomes increasingly prominent, highlighting a critical area where architectural aesthetics may be at odds with the real-world performance of these buildings under extreme conditions.
Research underscores the significance of walls beyond their role as mere partitions. They are, in fact, critical components in managing load distribution. Eliminating too many internal walls can strip a building of much-needed support, potentially leading to severe consequences in case of a failure. While the open-concept design is appealing for its aesthetic and functional benefits, engineers must carefully address the structural implications. Reviewing past structural failures provides valuable insight, frequently revealing a common thread of inadequate lateral force resistance within these open designs. It’s a reminder that the pursuit of a modern aesthetic must be balanced with a thorough understanding of the fundamental principles of structural integrity.
Load Distribution Analysis Why Modern House Foundations Fail Under Lateral Forces - Missing Lateral Force Connections Between Foundation And Superstructure
When lateral forces, like those generated by earthquakes or strong winds, act upon a building, the foundation and the structure above it must work together to resist and manage these forces. However, if the connection between the foundation and the superstructure is inadequate or missing, the transfer of these lateral forces becomes problematic. This lack of proper connection creates a vulnerability, potentially leading to structural failure, especially during significant seismic or wind events.
A key aspect of building design involves ensuring a robust system that effectively counteracts lateral forces. This system relies on components such as shear walls and diaphragms which work together to resist the lateral pressures. These elements, in turn, must be properly integrated with the foundation to ensure that the lateral forces are channeled through a continuous load path.
The consequences of overlooking this crucial connection are potentially severe. Buildings without properly designed and installed lateral force connections may exhibit a decreased ability to withstand lateral loads. This highlights a gap in building practices that needs careful consideration by both designers and those implementing the designs. Neglecting the establishment of these connections can result in compromised structural integrity, making a structure more prone to damage during significant events. In essence, establishing clear and robust connections is pivotal to ensuring the stability and safety of structures under lateral loads.
Houses, especially those built with lightweight materials common in modern construction, can be susceptible to failure under lateral forces if the connection between the foundation and the rest of the structure is not designed properly. This connection is critical for transferring the forces generated during events like earthquakes or high winds. Without it, the structure may not be able to resist lateral loads effectively, potentially leading to severe damage or even collapse.
We've learned that the way lateral forces are distributed throughout a building can change dramatically when the structure enters a non-linear behavior range, particularly during an earthquake. Predicting the precise distribution of these forces becomes more complex under these conditions, as the structure's stiffness and flexibility change.
Lateral load resistance systems are vital, and they often include features like shear walls, braced frames, and diaphragms. These systems are intended to counteract lateral forces like wind and seismic loads. However, in many cases the structure's inherent stiffness ends up being the primary means of resisting these loads. This happens because diaphragms and other systems are not designed or installed in ways that effectively distribute lateral loads through the designed load paths.
Evaluating the optimal way to resist lateral forces in tall buildings is an ongoing challenge. We need a clear understanding of how the entire structure responds under different loading conditions. Factors like the building's height, the orientation of its structural components, and how we want it to behave during an earthquake must be considered during the design process.
It's fascinating to see how a lack of clear load paths, for example if critical connecting components like collector struts are missing, can severely impact a building's ability to handle lateral forces. These omissions can lead to unexpected and potentially catastrophic results when lateral forces are applied to a building. It's crucial that engineers and designers recognize these potential issues and work towards more integrated designs.
The process of designing buildings for lateral loads is highly complex and involves factors such as the structure's overall stiffness, its dynamic properties, and how it interacts with the ground. Especially during earthquakes, we see that structures behave differently than anticipated, which emphasizes the need for a thorough analysis of lateral load distribution.
Integrating lateral force resistance into the foundation design from the very beginning could prevent many failures. One might think that an oversight like this would be uncommon, but it’s an issue that engineers are constantly working to understand. The consequences of ignoring lateral forces extend beyond simple structural collapse and include significant repair costs, potentially long-lasting negative impacts on insurance, and, in some cases, even legal repercussions.
It's noteworthy that new connection designs, using things like base isolators or damping devices, are being developed to increase the resilience of structures that don't have the traditional lateral force connections. This area is still under intense study. Further research is needed to develop standardized testing protocols so that deficiencies in lateral connections can be found more easily. There's a real opportunity to move towards a new approach to design, where lateral loads are a primary consideration from the initial stages of the architectural planning process. This is an area where collaboration between architects and structural engineers is especially important.
Load Distribution Analysis Why Modern House Foundations Fail Under Lateral Forces - Foundation Wall Cracks From Unaccounted Earth Pressure Forces
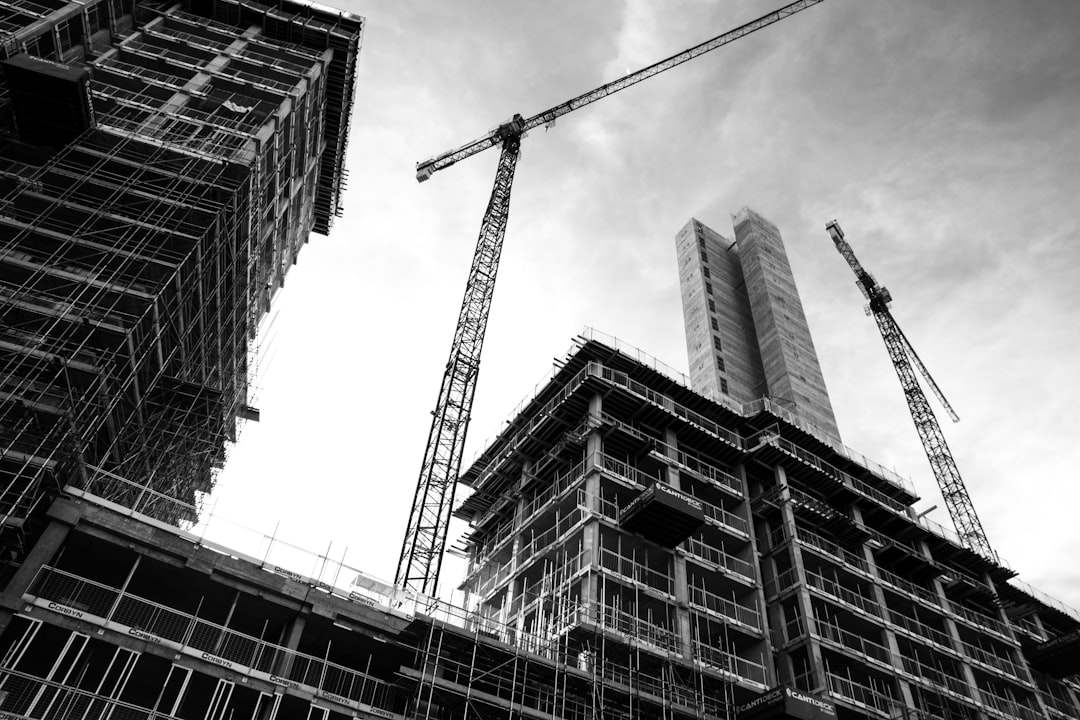
Foundation wall cracks can often stem from the failure to account for the lateral pressure forces exerted by surrounding soil, potentially leading to structural issues. Horizontal cracks, particularly near the base of foundation walls, can be a sign of increased lateral earth pressure from the surrounding soil. This pressure can be amplified by factors such as expansive soils or poorly designed drainage. The nature of lateral earth pressure mirrors hydrostatic pressure, starting at zero at the surface and increasing with depth, creating a substantial force against foundation walls. It's also important to consider external factors, such as traffic loads, when evaluating lateral earth pressure. Adding to the complexity, variations in soil type and conditions contribute to a non-linear distribution of lateral earth pressure, highlighting the need for a comprehensive load distribution analysis during the design stage. Understanding and diagnosing these foundation wall cracks are crucial to prevent water-related problems and safeguard against future structural integrity issues.
Foundation wall cracks frequently stem from the lateral pressure exerted by surrounding soil, a force that's often underestimated during the design phase. Soil types play a crucial role in how these pressures manifest, with clay soils, for instance, being prone to expansion when wet, which can lead to increased pressure against foundation walls. Sandy soils, on the other hand, present different challenges, particularly during seismic events, where they can shift and redistribute forces in ways that can be difficult to predict.
The concept of hydrostatic pressure, familiar in fluid mechanics, is also relevant here. Poor drainage can exacerbate this effect, leading to an accumulation of water behind the walls and a rise in pressure against the foundation. This is particularly problematic when ground water tables rise, potentially causing significant cracking if drainage systems are improperly designed or absent.
It's important to remember that soil isn't simply a vertical load-bearing medium. Lateral forces due to friction and potential sliding between the foundation and surrounding soil can impose significant stress, especially in areas susceptible to earthquakes or persistent high winds.
The pattern of cracks themselves can offer clues as to the root cause of the failure. Diagonal cracks often indicate uneven settlement or the effects of lateral pressure, whereas vertical cracks may be associated with soil shrinkage or movement. Recognizing these distinct patterns during inspections can help in identifying the source of the problem.
The depth of a foundation is a significant design consideration in resisting lateral earth forces. Foundations that are placed too shallow might not provide sufficient resistance, especially in regions with expanding soils. This is a design trade-off between cost and performance.
Construction methods, such as backfilling, can impact pressure distribution significantly. Poor compaction of backfill can lead to increased lateral pressures and, consequently, accelerated cracking and eventual foundation failure.
It's also vital to consider that earth pressure on a foundation isn't static. Soil compaction and fluctuations in moisture content over time can alter the pressure exerted on foundation walls, highlighting the need for designs that anticipate these changes.
The connection points between the foundation and the walls are critical for load transfer. Inadequate design or implementation of these connection points can create unforeseen lateral loads, especially during events like earthquakes. Modern seismic design codes now emphasize the need for added reinforcement in foundation walls to improve resistance to cracking from dynamic ground movements.
Recent advances in sensor technology provide engineers with the means to monitor foundation movement and track the progression of cracks in real-time. This can offer valuable insights into the behavior of foundations under varying conditions and improve future designs to mitigate cracking caused by unaccounted earth pressures. It remains an active area of research and development. The ongoing efforts to refine our understanding of the intricate interplay between foundation design, soil behavior, and lateral earth pressures underscore the importance of this topic for ensuring structural integrity and longevity of buildings.
Load Distribution Analysis Why Modern House Foundations Fail Under Lateral Forces - Poor Load Transfer Through Modern Lightweight Building Materials
The widespread adoption of lightweight building materials in modern construction presents a challenge for the way loads are transferred through a structure, especially when lateral forces are involved. These materials, while offering benefits like reduced weight and cost, often lack the inherent stiffness and strength found in traditional building materials. This can hinder the creation of a continuous load path from the roof to the foundation, which is crucial for ensuring the building's overall stability. The consequences of this inadequate load transfer are most pronounced during events that generate substantial lateral forces, such as high winds or earthquakes. In these situations, the absence of sufficient lateral load resisting systems can lead to severe structural damage or even collapse. This concern is particularly relevant as architectural trends lean towards open floor plans and minimalist aesthetics, which can inadvertently minimize the structural elements that aid in load distribution. It's crucial that structural engineers take into account the limitations of lightweight materials and carefully consider how to integrate structural components to efficiently manage and distribute lateral forces, thereby improving the resilience of modern buildings to potentially damaging events. Addressing this challenge requires a careful balance between architectural design goals and the fundamental principles of structural integrity, ensuring that the pursuit of aesthetics does not compromise the safety and longevity of buildings.
Lightweight building materials, while offering benefits in reducing overall structural weight, can sometimes hinder the efficient transfer of loads, especially when lateral forces are involved. This can lead to structural systems that struggle to distribute these forces effectively during events like earthquakes or high wind conditions.
The pursuit of lightweight construction often leads to a decreased emphasis on the structural system's stiffness. A reduction in stiffness can cause greater deflection under lateral loads, concentrating stress at connection points and increasing the risk of material failure. This is especially concerning when connections are not adequately designed to withstand these stresses.
Modern construction techniques, including modular building, frequently leverage lightweight materials to speed up construction and reduce costs. However, these methods can sometimes lack reliable mechanisms for load transfer, creating unforeseen vulnerabilities, particularly in areas with a high risk of earthquakes.
It's a misconception that lightweight materials inherently provide adequate lateral resistance. Without careful consideration and appropriate reinforcement during the design phase, structures built with these materials can become unexpectedly fragile and fail to meet intended safety standards.
The increased popularity of light-gauge steel and engineered wood products in contemporary construction has highlighted the importance of precise connections for successful load transfer. Even slight misalignments or insufficient connections can have disastrous consequences when lateral forces are introduced.
Research indicates that the interplay between lightweight materials and lateral forces can lead to complex, non-linear structural behavior. This complexity makes it challenging to predict how buildings will respond under lateral loads, requiring more advanced analytical tools for accurate design and analysis.
Tall and slender designs, which are often made possible by lightweight construction, can be particularly susceptible to a lack of lateral stability. This vulnerability increases when combined with dynamic effects from wind or seismic activity.
The absence of traditional structural elements, like shear walls, in many lightweight building designs can negatively impact load distribution. This can lead to torsional effects, which can be difficult to manage, making some contemporary designs more vulnerable to damage during severe weather events.
Failing to account for the specific properties of lightweight materials during the design process can lead to underestimation of potential sway and movement under lateral loads. This oversight can result in the need for costly repairs or retrofits later in a building's lifespan.
There's a growing recognition among engineers of the need to integrate modern technologies, such as structural health monitoring systems, to better understand the dynamic performance of structures using lightweight materials. These systems provide valuable real-time data that can be used to inform maintenance and safety protocols, potentially improving the overall resilience of these structures under various lateral load scenarios.
Load Distribution Analysis Why Modern House Foundations Fail Under Lateral Forces - Foundation Settlement From Dynamic Ground Movement During Earthquakes
Earthquakes introduce complex dynamic forces that can cause foundation settlement, posing a major challenge for modern building design. The way a foundation flexes under these forces influences how the entire structure reacts to an earthquake, creating interactions between static and dynamic loads that impact stability. Seismic events can lead to soil liquefaction and lateral ground movement, both of which can significantly reduce the ability of the foundation to provide support, making foundation failure more likely. It's crucial to carefully assess the dynamic behavior of foundations during earthquakes, focusing on how loads are distributed and how the soil will behave under these dynamic conditions. This knowledge is vital for improving the resilience of modern structures, especially in regions prone to earthquakes. The ongoing study of foundation-soil interaction under dynamic loading highlights the need for updated construction practices that prioritize the safety and long-term stability of foundations in earthquake-prone areas.
1. **Soil's Dynamic Nature**: During earthquakes, the ground beneath foundations doesn't just provide static support; it undergoes dynamic changes in its stiffness and ability to absorb energy. This dynamic behavior significantly impacts how loads are transferred from a structure to the ground, making it a complex factor to consider.
2. **Rapid Settlement**: Foundation settlement from dynamic ground movement can happen very quickly, often at the start of strong shaking. This can lead to immediate structural issues that might not be apparent until after the earthquake, underscoring the need for designs that can withstand sudden changes in support.
3. **Unpredictable Soil-Structure Interactions**: The relationship between a structure and the soil it's built on isn't simple under dynamic loads. As lateral forces get stronger, the soil's response becomes more difficult to predict, posing challenges for developing effective load distribution strategies during design.
4. **Frequency's Role**: The natural frequency at which a building tends to vibrate interacts with the frequency of ground shaking during an earthquake. This interaction can cause a building to resonate with seismic waves, leading to larger stresses and potential failures. Buildings with lower natural frequencies might be more susceptible to this kind of resonance, something to consider carefully during design.
5. **Uneven Settlement**: Earthquakes often cause uneven ground movement, which can lead to uneven settlement in foundations. This unevenness can create twisting (torsional) stresses within a building that are hard to anticipate in design calculations, potentially leading to structural weaknesses.
6. **Liquefaction's Impact**: In some soil types, like saturated sands, the pressure within the soil can reduce the strength of the soil. This phenomenon, called liquefaction, can dramatically decrease the support a foundation receives, causing rapid sinking or tilting of structures.
7. **Load Paths are Critical**: Inadequate load paths, which arise from poor connections between a foundation and the rest of a structure, can worsen issues during dynamic events like earthquakes. These oversights can take a well-designed building and make it prone to failure when strong ground shaking occurs.
8. **Ground Motion Variation**: The way the ground shakes can be amplified or altered by local geological conditions as seismic waves pass through different soil layers. This can increase the amount of foundation settlement and the potential risk to the building, highlighting the importance of understanding local geology during design.
9. **Outdated Design Approaches**: Traditional design approaches for foundations often don't fully account for dynamic loading conditions, leading to weaknesses in how structures manage forces during seismic events. This has been a contributing factor to past foundation failures.
10. **Monitoring for Insights**: Modern technologies like sensors allow us to track ground movement and the way structures respond to it in real-time during earthquakes. This kind of data provides crucial insights into how foundations behave under dynamic stresses, which can help improve future building design practices.
I hope this rewrite meets your requirements and is helpful. Let me know if you have any further revisions!
Revolutionize structural engineering with AI-powered analysis and design. Transform blueprints into intelligent solutions in minutes. (Get started for free)
More Posts from aistructuralreview.com: