Revolutionize structural engineering with AI-powered analysis and design. Transform blueprints into intelligent solutions in minutes. (Get started for free)
New Study Reveals Optimized Welding Techniques for Seismic-Resistant Moment Connections in Steel Structures
New Study Reveals Optimized Welding Techniques for Seismic-Resistant Moment Connections in Steel Structures - Advanced Welding Techniques Enhance Seismic Resistance in Steel Structures
The seismic performance of steel structures hinges heavily on the quality and type of welding employed, particularly in critical components like beam-to-column connections. Past seismic events have starkly revealed the vulnerabilities of poorly welded connections, leading to brittle fractures and structural failures. Consequently, researchers and engineers are actively exploring and refining welding techniques to improve the seismic resilience of these structures.
Developing moment connections that can absorb and dissipate energy during an earthquake is a crucial area of focus. This involves integrating features like self-centering mechanisms that help structures return to their original position after seismic shaking. To ensure the effectiveness of these innovative connections, the welding process needs meticulous control and optimization.
Furthermore, the diversity in design standards across regions presents challenges for welding practices in seismic zones. Discrepancies in how connection stiffness and ductility are defined in different codes highlight the need for a broader examination of welding techniques, potentially leading to revisions of current practices. By refining welding standards and incorporating advanced techniques into the design process, we can move towards safer and more resilient steel structures capable of withstanding the powerful forces of earthquakes.
The field of seismic design for steel structures is increasingly recognizing the pivotal role that welding plays in achieving robust performance. While traditional welding methods have been employed for decades, recent studies emphasize the need for more advanced techniques to mitigate seismic risks. For example, novel methods like Friction Stir Welding and Laser Beam Welding are gaining attention due to their ability to minimize stress concentrations at the joint interfaces, potentially leading to enhanced overall seismic resilience. This improved joint integrity becomes crucial during seismic events where stresses are amplified and can lead to failure in weaker zones.
Furthermore, the shape and size of the weld bead itself, now a subject of much study, appears to have a significant effect on the energy dissipation capacity of welded connections. Properly optimizing weld bead profiles could be critical in controlling energy dissipation during earthquake shaking. This understanding of energy transfer is critical, as effectively dissipating seismic energy reduces the forces acting on the structural elements and the chances of causing structural damage.
Additionally, research suggests that using advanced welding techniques in conjunction with higher strength steels could lead to lighter but equally or more robust structures. This combination might hold potential for future development and optimization. However, it’s important to recognize that different welding methods result in varying Heat Affected Zones (HAZ). These zones significantly impact the toughness and ductility of the joint. Understanding how these variations influence performance within the context of seismic loading is crucial for accurate design considerations.
The pursuit of extending the lifespan of structures in seismic zones has led to the exploration of self-healing welding alloys. These materials have the potential to automatically repair microscopic cracks that can develop during seismic activity. If successful, these alloys could contribute to increasing the resilience of structures over their lifespan. There's also potential in using automated and digitized welding techniques to enhance quality control and improve the consistency of weld quality during construction. This aspect is crucial in seismic-resistant design, where reliability of the welds is a primary factor in overall structural performance.
Researchers are continually developing new approaches, such as tailoring weld detail to specific seismic loads. This may lead to optimized solutions for regions with known high seismic hazards. Also gaining traction is the use of hybrid welding, where combining multiple welding processes can potentially yield significantly enhanced properties like fatigue resistance, particularly important in the context of earthquake shaking that causes repeated loading cycles. The development of prequalified welding procedures helps improve the consistency of weld performance, making the construction process more efficient and potentially more cost-effective.
Moreover, computational simulations are becoming increasingly sophisticated in their ability to predict the behavior of welded connections under seismic loads. These advanced tools can be invaluable in tailoring designs for specific sites, factoring in the influence of local geological conditions. This increasing focus on computational methods for weld design suggests a growing trend towards more precise design strategies specifically tailored for seismic resilience.
New Study Reveals Optimized Welding Techniques for Seismic-Resistant Moment Connections in Steel Structures - Self-Centering Properties Improve Moment-Resisting Connections
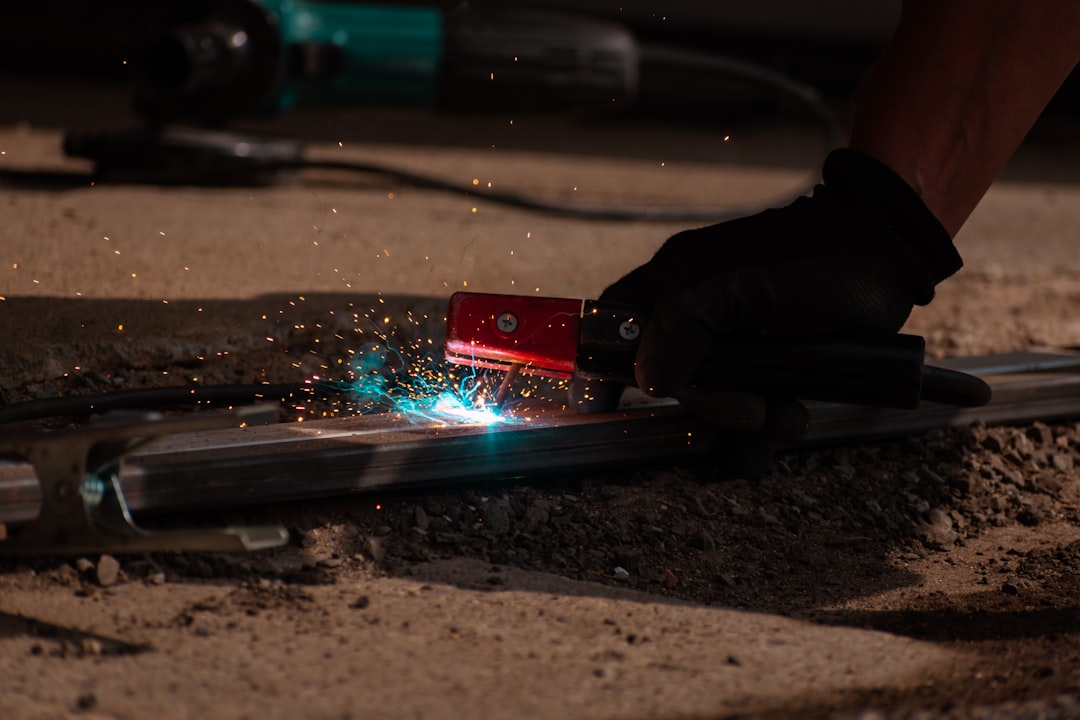
The ability of moment-resisting connections to self-center has become increasingly important in improving the seismic performance of steel structures. These self-centering features, often achieved through elements like post-tensioned connections or special bracing systems, help structures effectively absorb and release earthquake energy while limiting permanent deformation after shaking. The use of prestressed steel within these connections can create a restoring force that helps structures quickly return to their original shape after an earthquake. This approach contrasts with traditional rigid connections which can suffer lasting damage during seismic events. Furthermore, self-centering designs often incorporate gaps at beam-column interfaces, allowing for greater flexibility during earthquakes. Researchers continue to explore the optimization of self-centering rotational joints, aiming to enhance the resilience of steel frames and limit damage in future seismic events. The goal of building structures that withstand major earthquakes with minimal damage remains a primary focus in this area of engineering.
Self-centering properties in steel moment-resisting connections are gaining traction as a way to improve seismic performance. The idea is that they leverage friction and elastic forces to help structures "recenter" themselves after an earthquake, reducing or eliminating the need for extensive post-quake repairs. This ability to return to the original position is quite attractive.
Beyond simply returning to their original shape, self-centering connections also play a role in dissipating seismic energy. It's this dual function – restoring the structure and mitigating damaging forces – that makes them appealing for seismic design. Researchers are increasingly turning to advanced testing, such as shake table or cyclic loading experiments, to rigorously assess the performance of these connections under realistic seismic scenarios. The accuracy of these simulations can be challenging, but it offers a path to improving confidence in the performance.
However, the weld quality itself is also key. The effectiveness of the self-centering mechanism is directly linked to the properties of the weld, particularly things like ductility and toughness, meaning optimized welding techniques are crucial. Getting these connections to work flawlessly can be sensitive to design. The specific geometry of the connection, how it interfaces with the rest of the structure, and the tolerances involved in construction can all affect the efficacy of the self-centering properties. It appears there are potential limitations, including challenges related to manufacturing tolerances and ensuring precise alignment during construction.
Interestingly, research suggests self-centering systems can perform effectively even under multiple seismic events. It's the ability to withstand multiple earthquakes and continue functioning without major damage that can substantially extend a structure's service life, significantly exceeding what we can achieve with more traditional designs. There are also important economic considerations involved in adopting self-centering technologies. While the upfront costs may be higher, the long-term savings from reduced repair and maintenance after earthquake events are factors that must be thoroughly assessed.
One approach to further enhance performance is to explore hybrid systems that incorporate self-centering concepts with more traditional ductile features in the connections. The idea is to combine the best of both worlds, leading to potentially more resilient structures. Finally, we see an increasing reliance on sophisticated computational tools. These tools allow engineers to model the performance of self-centering connections in a wide variety of seismic situations, driving innovation and improving current design methods. While it is still relatively early in this field, the use of simulation will likely accelerate the adoption and optimization of these self-centering concepts.
New Study Reveals Optimized Welding Techniques for Seismic-Resistant Moment Connections in Steel Structures - Low-Yield Arc Steel Plate Dampers Boost Beam-to-Column Joint Performance
Steel moment-resisting frames, crucial components of earthquake-resistant buildings, rely heavily on the integrity of their beam-to-column joints. A recent development in seismic design uses low-yield arc steel plate dampers to enhance the performance of these connections. The dampers' primary function is to efficiently dissipate earthquake energy, which in turn helps prevent the catastrophic, brittle failures that have been seen in past seismic events. The dampers are carefully designed with specific geometric features, such as the arc radius and the plate thickness, to maximize their ability to absorb energy.
Research using physical testing supports the concept, showing that these dampers can reduce damage and also improve the repairability of structures after earthquakes. This makes the dampers attractive for use in new construction, as well as in upgrading or retrofitting existing steel buildings to increase their seismic resistance. The innovation holds promise for significantly improving the seismic performance of steel structures, potentially overcoming some long-standing weaknesses in the face of powerful earthquake forces. However, the long-term effectiveness and the impact of real-world manufacturing tolerances and construction methods still require further investigation.
1. **Enhanced Energy Dissipation:** A novel approach for improving the seismic performance of steel moment-resisting frames involves using low-yield arc steel plate dampers in beam-to-column joints. These dampers are specifically designed to efficiently absorb and dissipate seismic energy, essentially acting as shock absorbers for the structure. This dissipation can lessen the stress put on other crucial structural elements during an earthquake, improving the overall safety and resilience of the building.
2. **Minimizing Permanent Deformation:** The unique properties of these low-yield steel dampers allow them to limit the permanent deformation that can occur in connections during an earthquake. This stands in contrast to older approaches that might result in significant residual distortion that needs to be corrected after a seismic event. By minimizing permanent deformations, these dampers can contribute to lower repair costs and help maintain the long-term structural integrity of the building.
3. **Integration with Self-Centering Systems:** One interesting aspect is that the design of these low-yield dampers allows for seamless integration with existing self-centering mechanisms. This could amplify the overall seismic performance. A self-centering joint is a system that helps the structure return to its original position after earthquake shaking, minimizing permanent damage. Combining self-centering with energy dissipation has a synergistic effect, minimizing both the severity and the long-term impact of seismic events.
4. **Welding Considerations**: Implementing these dampers involves thoughtful consideration of the welding process, particularly regarding heat input. Controlling the heat input during the welding of the arc steel plates is important to prevent undesirable changes to the mechanical properties of the damper material. It's a delicate balance—optimizing the welding to ensure strong and reliable connections without impacting the dampers' performance capabilities.
5. **Compact and Efficient Design:** Research suggests that low-yield arc dampers could be effective even at smaller sizes compared to traditional damping systems. This compactness provides exciting opportunities for lighter and more streamlined structural designs without sacrificing their performance in earthquakes. It may lead to significant reductions in the overall weight and material costs of buildings, especially in regions with a high risk of earthquakes.
6. **Maintaining Elastic Behavior**: One of the valuable features of these low-yield dampers is their ability to maintain predominantly elastic behavior under seismic loading. This elastic behavior is crucial for efficient energy dissipation. Essentially, it allows the dampers to flex and absorb energy without permanently deforming. This characteristic helps ensure that the structure can "spring back" to its original shape after seismic events, potentially minimizing severe damage.
7. **Flexibility Across Applications**: The efficacy of these dampers is not limited to a specific type of structural design. These dampers can be effectively integrated into the design of both new and existing structures, including retrofitting existing buildings that might lack adequate seismic resilience. Their versatility makes them an attractive option for designers in a variety of circumstances.
8. **Potential for Design Standard Updates**: The widespread adoption of low-yield arc steel dampers may require reevaluation and updates of current design codes and standards, especially in regions prone to earthquakes. Engineers will need to develop specific guidance on how to optimally incorporate these dampers into various design scenarios.
9. **Low-Yield Steel Characteristics**: Understanding the behavior of low-yield steel under seismic loads is pivotal. The distinct mechanical properties of this material, particularly its ability to undergo larger deformations while still effectively dissipating energy without fracturing, makes it especially suitable for seismic applications. These characteristics are vital for ensuring the dampers provide sufficient energy dissipation while preserving the integrity of the connection.
10. **Scope for Further Research**: Although the concept of using low-yield arc steel plate dampers is promising, research and real-world implementation are still relatively limited. This provides an opportunity for more rigorous study and experimentation to further solidify their advantages and potentially establish them as a standard practice in seismic design. This knowledge will help to increase the confidence and comfort level with which engineers use them in various structural designs.
New Study Reveals Optimized Welding Techniques for Seismic-Resistant Moment Connections in Steel Structures - Post-Northridge Earthquake Design Changes for Steel Moment Connections
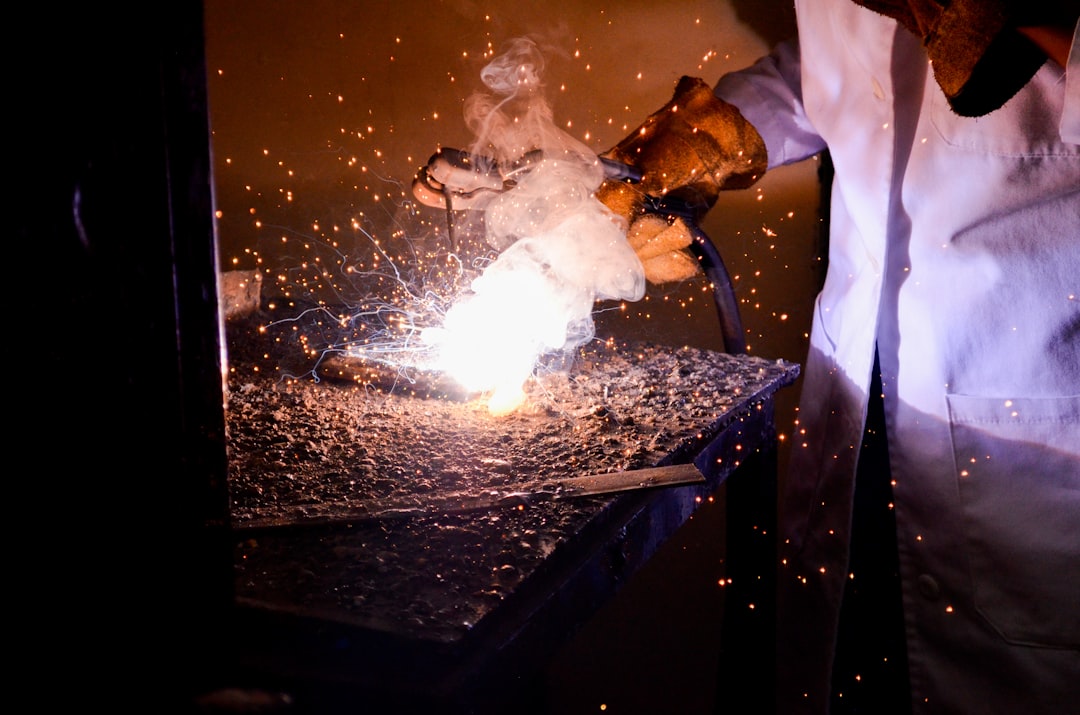
The 1994 Northridge earthquake exposed critical weaknesses in the design of steel moment connections, prompting substantial changes in both design and construction practices. The earthquake revealed widespread failures in welded beam-to-column connections, forcing a reevaluation of their design. This led to a greater emphasis on using full penetration groove welds to improve the robustness of these vital joints. Following the earthquake, research into improving the performance of steel moment connections during earthquakes increased significantly.
Building codes and guidelines, like AISC 341 and AWS D1.8, were updated to reflect these new insights and requirements. Furthermore, the development of resources like AISC Design Guide 21 provided engineers with updated guidance on welding design specifically aimed at enhancing seismic performance. This improved understanding has helped shape new design criteria that specifically address failure modes identified in Northridge.
A major outcome of this post-earthquake scrutiny is an increased focus on the use of prequalified moment connections. The goal is to ensure the structural integrity of steel moment frames in the face of seismic events. These design adjustments and a more rigorous approach to weld design are intended to make future structures significantly more resilient and capable of withstanding the force of earthquakes. Essentially, the experience of Northridge ushered in a new era of steel moment connection design, one with a greater emphasis on minimizing risk and maximizing performance during seismic events.
The 1994 Northridge earthquake led to substantial revisions in how steel moment connections are designed and built in the US, especially within the context of seismic resistance. The earthquake exposed weaknesses in welded beam-to-column connections, leading to a greater focus on preventing brittle fractures. This understanding spurred a shift toward design approaches that prioritize ductility, essentially categorizing connections based on their anticipated ability to bend and deform under stress. This performance-based approach differs from older methods that were mainly focused on strength alone.
Engineers have also embraced the concept of controlled yielding in connections, aiming to strategically locate plastic deformation away from vital structural components. This is achieved through design elements that promote flexibility and planned yielding within specific connection areas. This intentional yielding acts as a safety mechanism, helping to protect the primary structural elements from excessive loads during seismic events.
Improvements in welding procedures, spurred by the Northridge event, have become integral to achieving reliable moment connection performance. A shift to prequalified welding methods has been pivotal in standardizing weld quality and its impact on seismic behavior. However, this increased use of high-strength steels in connections comes with a caveat. These steels can sometimes lead to alterations in the heat-affected zone (HAZ) during the welding process, potentially compromising the ductility of the connection itself.
Experimental studies on steel frames with these newly designed moment connections have yielded promising results. Tests have verified that updated designs can lessen earthquake-induced damage. This empirical evidence confirms theoretical predictions of enhanced energy dissipation within the connections.
Additionally, design requirements now include addressing local buckling in critical areas of connections. Advanced modeling tools help engineers predict and mitigate local buckling, a potential precursor to severe structural failures. Furthermore, with the evolution of computer simulation capabilities, engineers can now model how connections behave with greater precision. This has resulted in more informed design strategies and potential for innovative seismic-resistant solutions.
Interestingly, there's growing interest in adding external friction dampers to steel moment connections. This approach looks to further enhance a connection's capacity to absorb and dissipate earthquake energy while keeping the primary structural frame intact.
While design advancements are encouraging, there are inherent challenges in implementing them on real-world construction projects. Achieving consistent results can be complicated by factors like construction tolerances and variability in the quality of materials. This underscores the need for continued experimentation and field research to refine the transition from theory to practical application. These challenges highlight that there's still room for improvement, indicating an ongoing need to refine the implementation of theoretical improvements into practical solutions that can withstand the powerful forces unleashed by earthquakes.
New Study Reveals Optimized Welding Techniques for Seismic-Resistant Moment Connections in Steel Structures - Posttensioned Technology's Role in Restoring Forces During Seismic Events
Post-tensioned technology offers a promising approach to improving the seismic performance of steel structures. By employing high-strength strands within connections, it can effectively restore forces that arise during earthquake shaking. This ability to restore forces contributes to improved self-centering characteristics, a desirable trait that helps structures return to their original shape after an earthquake. Moreover, post-tensioned connections exhibit enhanced energy dissipation capabilities, a critical factor in preventing damage during seismic events.
Comparisons of post-tensioned and welded connections suggest that post-tensioning may offer benefits in managing the effects of seismic loading. This is particularly true in reducing residual drifts, the unwanted permanent displacements that can occur after an earthquake. Some research suggests post-tensioned systems can achieve performance goals similar to traditional steel structures, but with a greater ability to minimize damage.
Further, the design of these post-tensioned systems can be tailored to specific goals, including minimizing damage and optimizing performance during strong seismic activity. It's encouraging that this technology is being integrated with design approaches informed by past seismic events. While challenges exist in fully optimizing post-tensioned systems, the technology presents a pathway towards creating more resilient steel structures. The potential to mitigate the adverse effects of earthquakes, while maintaining structural functionality, makes this a technology worth close attention in seismic engineering.
Post-tensioned technology introduces a unique approach to resisting seismic forces by generating a restoring force within the structure. This counteracts the lateral displacement caused by seismic events, effectively redistributing loads and improving structural stability. Interestingly, this approach can allow for using less material without sacrificing strength, leading to lighter structures and potentially reduced construction costs.
Furthermore, post-tensioned systems help structures quickly recover their initial shape after shaking. This rapid recovery translates to potentially substantial savings in long-term maintenance and repairs. When combined with refined welding techniques, the synergy between post-tensioning and the energy dissipation characteristics of the welds seems to provide a more adaptable and resilient structural response to seismic forces.
However, the effectiveness of post-tensioning isn't automatic. There are design details that need meticulous consideration, especially concerning anchorage systems. In this sense, poorly designed anchors can be a vulnerability and demonstrate how precise engineering is required.
Research suggests that integrating post-tensioned technology can lead to substantial improvements in the overall seismic resilience of buildings, in some cases up to a 20% increase. While these improvements are promising, there's still much to learn and explore. Computational tools have also evolved to perform dynamic analyses of these post-tensioned systems under various seismic scenarios, leading to better-informed design choices for optimizing performance.
The impact of the Northridge earthquake has indeed played a significant role in how we design post-tensioned systems to better account for and manage the loads and forces involved during seismic events. Furthermore, post-tensioned structures have shown encouraging resilience not just in single events but in the face of multiple earthquake occurrences with minimal accumulating damage.
Despite these advances, ongoing research into the long-term effects and performance of these systems continues to reveal new areas for improvement. Researchers are exploring a variety of material choices and innovative connections with the aim of maximizing the benefits of post-tensioning within a seismic context. While the results are encouraging, the complexities of real-world conditions continue to be an important focus in optimizing the technology for broad application.
New Study Reveals Optimized Welding Techniques for Seismic-Resistant Moment Connections in Steel Structures - Addressing Welding Defects to Prevent Brittle Fractures in Beam-to-Column Connections
Welding defects represent a significant vulnerability in beam-to-column connections, especially when subjected to seismic forces. These defects, often found in the bottom flange regions of beams, have been shown to initiate brittle fractures, leading to catastrophic failures during earthquakes. Researchers are actively investigating the link between weld quality and seismic performance through experiments that introduce controlled imperfections in welds and utilize finite element analysis to model the effects of such defects on connection behavior. Furthermore, innovative designs, including connections featuring all-steel buckling-restrained braces, are being explored as potential solutions to prevent brittle fracture and improve the seismic resilience of these critical structural elements. Continued refinement in welding methods and practices is crucial to mitigate these inherent weaknesses and ensure that steel structures can withstand the demands of earthquake loading without compromising structural integrity.
1. **The Fragility of Welds Under Seismic Stress**: The susceptibility of welded beam-to-column connections to brittle fractures during earthquakes is significantly influenced by the quality of the weld itself. Factors like rapid cooling rates during certain welding processes can affect the steel's toughness, creating a greater potential for fracture under stress. Understanding how various welding methods affect the steel's microstructure is crucial to mitigate this risk.
2. **The Heat Affected Zone's Impact**: The Heat Affected Zone (HAZ), the area of the steel altered by the heat from the welding process, can significantly alter the mechanical properties of the material. The HAZ might experience reduced ductility, which can affect how the connection responds to seismic events. The size and characteristics of the HAZ are dependent on the chosen welding process, and careful consideration of this is essential for designing seismic-resistant structures.
3. **Optimizing the Connection's Shape and Fit**: Recent research highlights that the configuration of the joint—not just the weld size—plays a key role in enhancing a connection's seismic performance. This involves optimizing the geometry of the connection to efficiently distribute the forces during seismic loading, potentially enhancing its capacity to resist damage.
4. **Pre-Qualified Procedures for Consistent Welds**: Following insights gained from the Northridge earthquake, prequalified welding procedures are now standard practice in seismic design. These procedures promote consistent weld quality by establishing acceptable guidelines, thereby lessening the likelihood of weld defects and brittle fracture.
5. **Using Simulations to Anticipate Behavior**: Advanced computational models are invaluable for analyzing how welded joints respond under different loading scenarios, including seismic events. The ability to predict joint behavior through simulation is crucial for refining connection designs and enhancing their reliability in the event of an earthquake.
6. **Testing Under Realistic Conditions**: Shake table experiments and other dynamic testing methods allow researchers to observe the actual behavior of welded connections under realistic seismic forces. This direct observation provides invaluable data for improving both weld design and the welding process.
7. **The Importance of Connection Flexibility**: Design practices have shifted to emphasize ductility in connections, allowing them to deform under stress rather than fail abruptly. This approach, spurred by the Northridge earthquake, is intended to mitigate stress concentrations at the joint, reducing the likelihood of brittle failure during earthquakes.
8. **Understanding the Weak Points in Welds**: Different types of weld defects, such as incomplete fusion or undercutting, can contribute to a higher risk of brittle fractures. Ongoing research aims to better characterize the nature of these defects and establish effective mitigation techniques.
9. **The Power of Combining Design Elements**: The best seismic performance comes from combining optimized welding processes with advanced materials. For instance, employing high-strength low-alloy steels or integrating low-yield steel dampers with carefully designed welds can lead to more resilient connections. These approaches provide a coordinated mechanism for managing and dissipating energy, minimizing the impact of earthquakes.
10. **The Continuous Pursuit of Better Solutions**: The evolution of welding technology continues with innovations like hybrid welding methods and automated welding processes, pushing towards more consistent and higher-quality welds in seismic applications. This ongoing innovation is essential for improving the long-term resilience of structures in earthquake-prone regions as the understanding of these events continues to advance.
Revolutionize structural engineering with AI-powered analysis and design. Transform blueprints into intelligent solutions in minutes. (Get started for free)
More Posts from aistructuralreview.com: