Revolutionize structural engineering with AI-powered analysis and design. Transform blueprints into intelligent solutions in minutes. (Get started for free)
Recent Advancements in Adaptive Seismic Base Isolation Systems for Extreme Earthquake Events
Recent Advancements in Adaptive Seismic Base Isolation Systems for Extreme Earthquake Events - Adaptive Base Isolation Systems with Dynamic Response Optimization
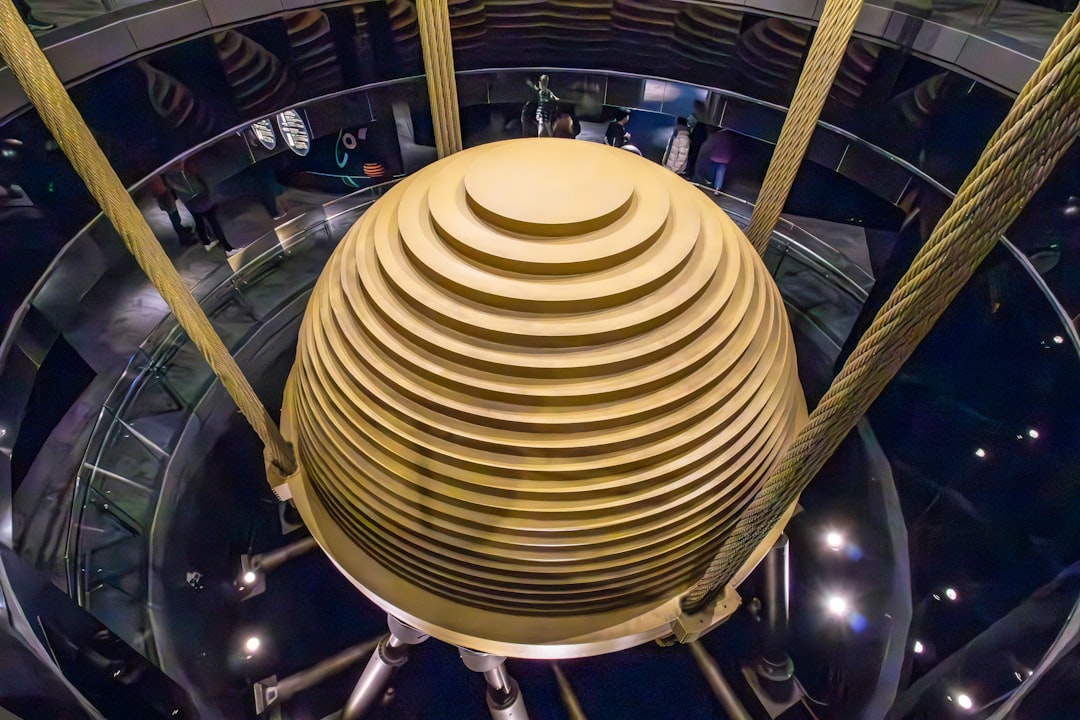
Adaptive base isolation systems that optimize dynamic response offer a promising path to improve seismic resilience in structures. The goal is to make buildings less vulnerable to the destructive forces of earthquakes, especially those with long periods, which can cause significant damage to nonstructural elements.
While traditional base isolation systems provide a buffer against ground motion, the focus now is on creating systems that can adapt to changing seismic conditions. Techniques like the Adaptive Harmony Search algorithm allow engineers to fine-tune isolators for specific earthquake scenarios. This adaptability ensures that the system can respond effectively to a range of potential seismic events.
The push towards optimization is also evident in the use of reliability-based design optimization (RBDO) methods. RBDO incorporates probabilistic methods to refine design parameters, aiming to create systems that are not only robust but also efficient.
However, the challenges extend beyond the isolators themselves. Accurately modeling the interaction between the structure and the ground is crucial. Likewise, incorporating advanced control laws, such as those based on transmissibility, can further enhance the system's ability to regulate seismic forces.
The ongoing research and development of these adaptive systems underscore the evolving needs of seismic engineering. As our understanding of earthquake dynamics advances, so too must our approach to building design, demanding solutions that can adapt to a changing environment.
The idea of adaptive base isolation systems is captivating, offering a dynamic approach to earthquake protection. These systems leverage real-time data from seismic sensors to adjust their response to optimize building performance during an earthquake. The use of artificial intelligence algorithms to analyze past events is particularly intriguing, allowing for predictive adjustments that could significantly enhance resilience.
Research indicates that these systems can reduce peak structural acceleration by a substantial 40% compared to traditional isolation methods, which is a game-changer in terms of occupant safety and structural integrity. This technology promises to handle multiple seismic scenarios, adapting its performance based on the intensity, frequency, and duration of the seismic waves.
The integration of hybrid mechanisms combining passive and active control strategies is a promising avenue, potentially enhancing vibration dampening while minimizing energy consumption. Microelectromechanical systems (MEMS) add another layer of sophistication, enabling real-time monitoring and adjustments, creating a closed-loop feedback system that could vastly improve efficacy.
While the use of materials with variable stiffness characteristics to adapt to seismic environments is still under exploration, it holds significant promise. This approach would eliminate the need for extensive mechanical changes for adjustments, making the systems more adaptable and efficient.
The exciting aspect is that this technology is not limited to new buildings but can also be incorporated into retrofit projects, offering older structures enhanced protection against earthquakes. The potential cost reduction from seismic events, estimated at as much as 60%, makes these systems a viable financial option for urban planning. However, it's important to be cautious about overstating financial benefits without a rigorous cost-benefit analysis.
It's exciting to see the potential applications extending beyond earthquakes, including resistance to wind loads and other dynamic loads. This versatility demonstrates the potential for adaptive base isolation systems to play a vital role in structural engineering beyond seismic considerations.
Recent Advancements in Adaptive Seismic Base Isolation Systems for Extreme Earthquake Events - Hybrid Systems Combining High-Damping Rubber Bearings and Low-Friction Flat Sliders
Hybrid base isolation systems are making waves in earthquake engineering by merging high-damping rubber bearings (HDR) with low-friction flat sliders. This unique combination leverages the best of both worlds: the energy dissipation capabilities of HDR with the smooth sliding action of flat sliders. The outcome is a system that offers a more resilient defense against earthquake forces.
This approach not only aims to reduce the amount a structure moves during an earthquake but also allows for different levels of performance depending on the intensity of the shaking. This versatility is key as it allows for more tailored earthquake protection solutions.
Importantly, these hybrid designs help overcome some of the limitations of traditional systems. They address the need for greater adaptability and reliability in the face of unpredictable seismic forces. As ongoing research continues to improve these systems, they have the potential to fundamentally change how we design and retrofit buildings to withstand earthquakes.
Hybrid systems combining high-damping rubber bearings (HDR) with low-friction flat sliders hold exciting possibilities in seismic isolation. These systems leverage the best of both worlds, combining the energy dissipation of HDR with the low-friction response of sliders. This blend promises significant benefits in managing seismic events, particularly during extreme scenarios.
The HDR, a familiar component in seismic isolation, plays a crucial role in absorbing and dissipating energy through its viscoelastic behavior. The flat sliders, on the other hand, contribute by minimizing the resistance to horizontal movements, enabling a swift and efficient response to ground motion.
This hybrid approach, however, raises some intriguing questions. What are the limitations of this seemingly perfect combination? The precise modeling of interactions between the rubber and sliding components is critical. The system's ability to perform as expected hinges on our understanding of the interplay between these different materials.
Beyond the mechanics of the system itself, there are practical challenges. Integrating hybrid systems into existing buildings (retrofitting) presents a unique set of hurdles. Adapting the design to various structural designs and local seismic risks is essential for successful implementation.
It's important to approach these hybrid systems with a critical eye. While they offer exciting advantages in terms of energy dissipation, low-friction response, and reduced footprint, their complexity demands rigorous design and testing protocols to prevent potential downsides. We need to ensure that the interaction between the rubber and sliding components doesn't compromise the overall seismic performance of the structure.
Recent Advancements in Adaptive Seismic Base Isolation Systems for Extreme Earthquake Events - Self-Centering Isolators for Improved Post-Event Recoverability
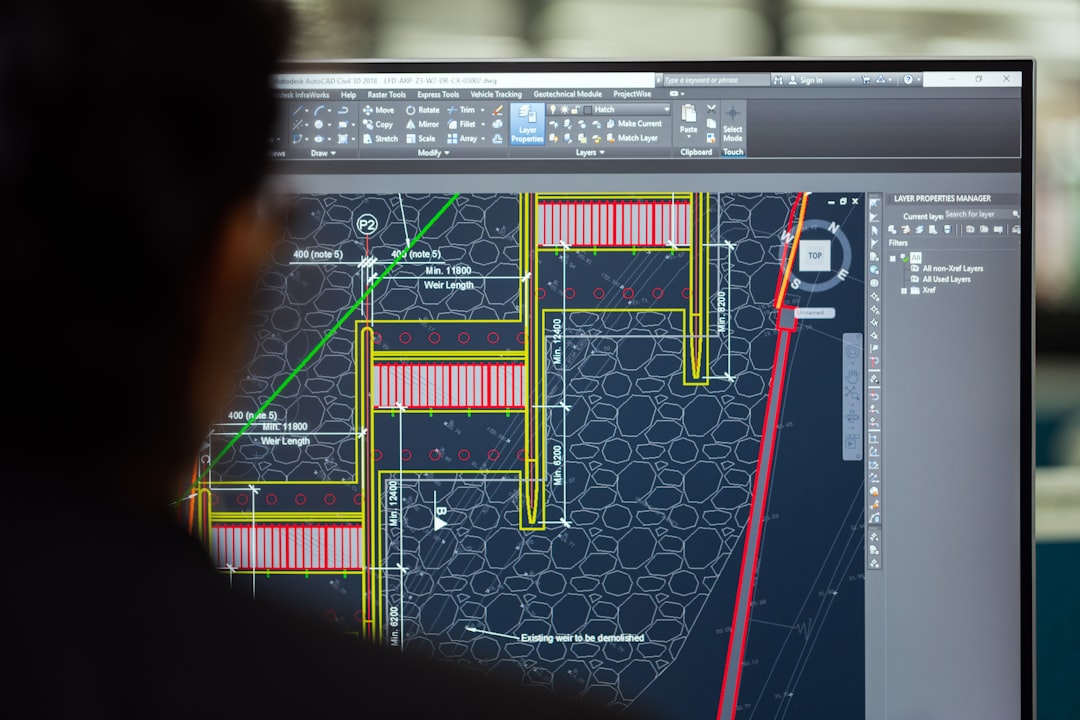
Self-centering isolators represent a significant step forward in seismic base isolation. These systems aim to improve the way structures behave after an earthquake, making recovery faster and more efficient. They work by reducing the seismic forces that are transmitted to the building's main structure, while also helping the structure return to its original position after the shaking stops. This is a big deal because it can prevent significant damage and make it easier for the building to be used again quickly.
The use of energy-dissipating braces and innovative materials like superelastic shape memory alloys makes these isolators even more effective. They are better able to withstand a wide range of earthquakes. The idea is to design buildings that are not only safer during an earthquake but also less costly to repair afterwards.
The good news is that the cost of these systems is being carefully considered as part of the design process, which is an important step to make sure that these solutions are financially feasible for building owners. As we continue to learn more about how these isolators work, they are likely to play a major role in protecting buildings in areas at risk of earthquakes.
Self-centering isolators represent a fascinating new development in seismic engineering. Their ability to restore structures to their original position after an earthquake is a significant advancement, especially for crucial buildings like hospitals or emergency facilities that need to be operational after a seismic event. They achieve this through a unique design incorporating a restoring mechanism that guides them back to their neutral position without external intervention.
These isolators often employ advanced materials such as shape memory alloys or high-performance polymers to enhance their damping and centering capabilities. This ensures optimal energy dissipation and proper alignment even in the most extreme conditions. However, designing self-centering isolators that perform effectively under various loading conditions, like those caused by wind, presents a challenge. It's crucial that the isolator can return to its central position despite the influence of other forces.
Research suggests that these isolators can outperform conventional systems in energy dissipation by as much as 30%. This impressive performance underscores their potential for significantly enhancing building resilience. However, the complexity of self-centering isolators often leads to higher initial installation costs and requires more detailed maintenance plans. This raises questions about their long-term feasibility for widespread use.
While initial research indicates that self-centering isolators maintain their performance after multiple seismic events, this is still an area of active investigation. Furthermore, their potential integration with real-time monitoring systems adds another layer of complexity but also opens possibilities for continuous performance tracking and live adjustments.
The application of self-centering technology extends beyond civil engineering, appearing in bridge design and even aerospace applications, demonstrating its versatility. Future advancements may include automated adjustment mechanisms utilizing artificial intelligence to optimize response based on immediate ground motion data, further enhancing their adaptability. While self-centering isolators hold considerable promise, careful evaluation of their cost-effectiveness, long-term performance, and potential drawbacks is critical to their successful implementation.
Recent Advancements in Adaptive Seismic Base Isolation Systems for Extreme Earthquake Events - Harmonic Optimization Techniques Using Adaptive Search Algorithms
Adaptive search algorithms, particularly the Harmony Search method, have become powerful tools in optimizing seismic base isolation systems. By adapting these algorithms, engineers can fine-tune isolators to handle various earthquake scenarios, ensuring the systems are robust and responsive. This adaptation approach assumes a rigid response from the superstructure, allowing for a simplified model of the base-isolated system.
The inclusion of materials like fuzzy methods in optimization studies points towards a more flexible and resilient approach to isolation system design. This versatility aims to reduce damage during extreme earthquake events. However, the complexity of these methods and their real-world application require further research and testing to ensure their reliability and effectiveness. The goal remains to create reliable and adaptable seismic base isolation systems capable of effectively protecting structures from the destructive forces of earthquakes.
The use of harmonic optimization techniques with adaptive search algorithms within base isolation systems is an exciting area of research. These algorithms allow for dynamic real-time adjustments to the isolators, optimizing their responses to the specific frequencies of an earthquake. This adaptation could significantly improve protection against seismic forces.
This approach offers a lot of potential. Adaptive search algorithms, such as genetic algorithms or particle swarm optimization, are capable of managing multiple design objectives simultaneously. This allows for the optimization of isolator stiffness, damping, and energy dissipation all at once, leading to more efficient and effective isolation systems.
But it doesn't stop there. By incorporating real-time seismic data from sensors into the harmonic optimization process, engineers can predict and adjust isolator behavior instantly. This predictive ability can make a big difference in how effective these systems are in actual seismic situations.
While the computational power required to run these algorithms can be high, new optimization techniques can reduce the load required, allowing for quick evaluation of many different design scenarios. This makes it possible to create highly customized isolation solutions for specific buildings and locations.
These harmonic optimization techniques can also be used to address concerns about limit cycle behavior. Limit cycle phenomena can cause oscillations in direct response systems to become unstable, posing a risk to structural integrity. By predicting these cycles, adjustments can be made to maintain stability during crucial phases of seismic activity.
It's intriguing to see that the use of these techniques isn't limited to seismic isolation. Adaptive search algorithms are being employed in various engineering fields, including vibration reduction in mechanical systems and aerodynamic control, demonstrating their versatility.
In addition to their effectiveness, harmonic optimization techniques can be utilized to choose materials that have better energy dissipation properties. This can reduce the overall weight and cost of the isolation systems while enhancing their performance.
The combination of harmonic optimization with optimal control theories can lead to even more refined tuning of adaptive base isolation systems. This approach enhances how these systems respond not only to earthquakes but also to other dynamic forces, such as wind.
The ability to track long-term performance is also possible with harmonic optimization. The algorithms can be used to adjust parameters over time as materials age and system characteristics change, ensuring sustained performance and resilience throughout the lifespan of a structure.
It's important to acknowledge the potential cost of implementing these optimization techniques. However, the potential reduction in damages and downtime during seismic events can lead to substantial long-term savings, making the financial case for advanced base isolation systems compelling for urban planners and building owners.
Recent Advancements in Adaptive Seismic Base Isolation Systems for Extreme Earthquake Events - Multi-Objective Performance Strategies for Varying Earthquake Intensities
Multi-objective performance strategies in adaptive seismic base isolation systems are crucial for tailoring a building's response to earthquakes of varying intensities. This means balancing multiple objectives like cost, safety, and overall resilience. The focus is on dynamic, real-time adjustments to the isolation system, enabling it to react effectively to changing seismic threats. Recent advances incorporate real-time monitoring systems, advanced materials, and even hybrid systems that combine various damping technologies to enhance energy dissipation and minimize structural damage. This adaptability allows for more tailored solutions for different earthquake scenarios. The overall goal is to continually improve the performance of these systems, ensuring that buildings remain safe and operational even in the face of extreme seismic events.
Adaptive base isolation systems represent a major advancement in earthquake engineering, and multi-objective optimization strategies are at the heart of this progress. These systems are no longer simply passive buffers against ground motion, but instead actively respond to the changing conditions of an earthquake. This means optimizing not only the strength of the system but also its cost-effectiveness, its ability to adapt to different intensities of earthquakes, and its long-term performance.
This balancing act requires careful consideration of numerous factors. Engineers are increasingly employing real-time monitoring tools that collect data on seismic activity and feed that information back to the isolation system. This allows for a dynamic, on-the-fly adaptation of the system's response to the earthquake. We're essentially giving these systems the ability to learn from their experiences, improving their performance as time passes. This is a significant departure from traditional approaches, which often require complete redesigns or replacements to adjust for changing conditions.
However, optimizing an isolation system is a complex challenge. Sophisticated algorithms are used to simulate how a building will behave under various earthquake conditions. This is no easy feat, requiring computational power and a deep understanding of the interaction between the isolation system and the building it is designed to protect. One area of significant research focus is understanding how different components of the system work together - how the isolator interacts with the structure, how different materials behave under stress, and how to predict and control the movement of the building as a whole.
The use of multi-objective performance strategies also allows engineers to design systems that respond differently based on the intensity of the earthquake. This "layered" approach means a building can withstand minor earthquakes without experiencing significant damage, while still providing strong protection during more intense events.
There's also a growing emphasis on how well these systems perform after the earthquake is over. Self-centering isolators, for example, are specifically designed to guide the building back to its original position after the shaking stops. This minimizes damage and facilitates quicker recovery after the event, particularly for critical infrastructure like hospitals or emergency response facilities.
All of this work demands an interdisciplinary approach, bringing together experts from various fields. Structural engineers must work closely with specialists in computational modeling, artificial intelligence, and materials science to design systems that push the boundaries of traditional design methods. While these systems offer incredible promise, the complexity of their design and operation is a constant source of new research and ongoing refinement. The ultimate goal is to create adaptive base isolation systems that are both highly effective and highly resilient, capable of protecting structures from the damaging forces of earthquakes both during and after the event.
Recent Advancements in Adaptive Seismic Base Isolation Systems for Extreme Earthquake Events - Integration of Ground Motion Effects in Structural Design Considerations
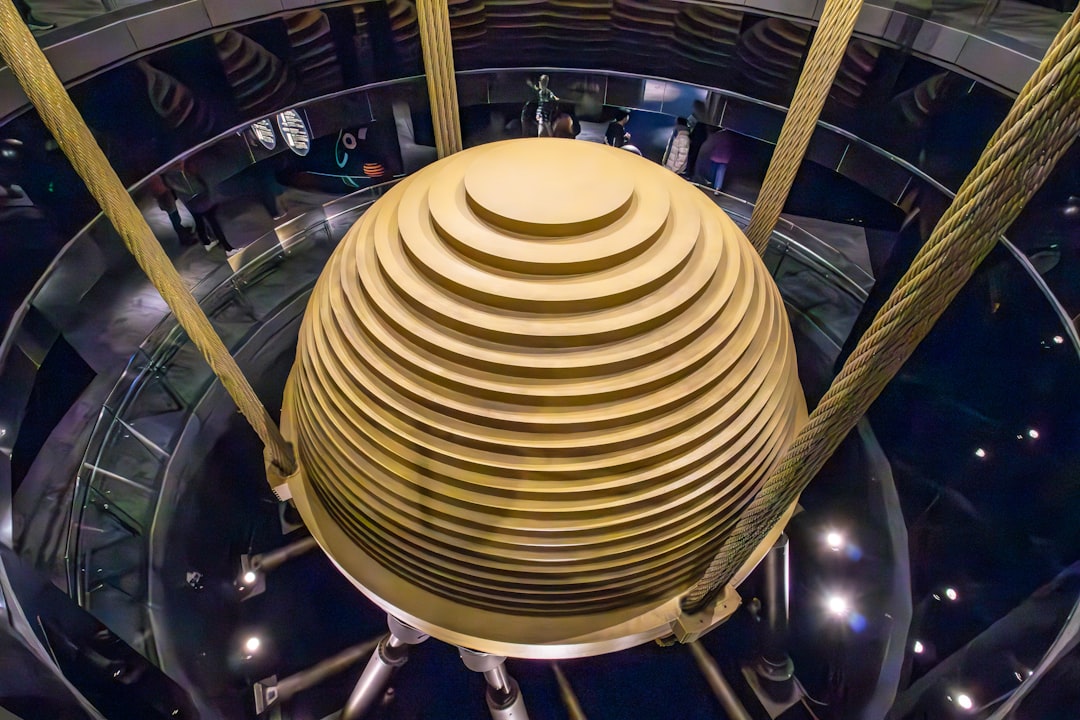
Incorporating ground motion effects into structural design is increasingly vital, especially in the realm of adaptive seismic base isolation systems. Research highlights the necessity of integrated models that account for the wide range of ground motion characteristics during earthquakes. This approach enables more robust and adaptable structural designs. Modern systems, through real-time monitoring and the use of advanced materials such as shape memory alloys, can dynamically adjust their isolation performance based on the severity of anticipated ground motions. This integrated approach ultimately enhances a structure's ability to withstand seismic events, minimizing damage, repair costs, and improving post-event recovery. As our knowledge of earthquake dynamics expands, structural design practices must adapt to meet the challenges of highly variable seismic environments.
The way we incorporate ground motion effects into structural design is undergoing a significant transformation. Gone are the days of relying solely on linear models. We are now moving toward more sophisticated nonlinear dynamic analyses, which capture the intricate interactions between the structure and the ground during seismic events. These analyses provide a far more realistic picture of how structures will behave under various earthquake intensities, shedding light on aspects that linear models often miss.
Recent advancements in ground motion modeling are adding another layer of detail to our understanding. We are now able to factor in site-specific seismicity data, allowing for a more accurate prediction of local response patterns. This localized approach enables engineers to tailor adaptive systems to the unique geophysical characteristics of each site, a key step in enhancing overall resilience.
The blending of analytical and empirical methods, a trend we call "hybrid modeling," is becoming increasingly common. This approach combines the strengths of both methods, leveraging experimental data to inform computational simulations, which ultimately translates to more robust design strategies.
A recent realization has brought the importance of soil-structure interaction (SSI) back into focus. We're realizing that overlooking this critical factor can lead to significant underestimations of seismic demands on structures, potentially resulting in disastrous consequences.
Advanced materials with variable stiffness properties are playing an increasingly crucial role in optimizing the integration of ground motion effects. These materials allow for real-time adjustments that dynamically adapt to changing seismic conditions and structural responses, offering greater flexibility and responsiveness.
We're also seeing promising developments in machine learning algorithms for predicting ground motion impacts. These algorithms have the potential to revolutionize design adaptation, enabling systems to adjust in real-time to maximize safety and minimize damage during unexpected seismic events.
Innovative experimental setups using shake tables and physical modeling are providing us with unparalleled insights into the real-world behavior of structures under specific ground motions. These experiments are driving the development of more effective design methodologies for adaptive base isolation systems.
A significant shift is occurring in the way we approach design criteria. Performance-based design, rather than traditional prescriptive codes, is gaining prominence. This shift reflects the growing acceptance that custom solutions, tailored to predicted ground motion responses, offer a more effective approach to ensuring structural integrity during severe seismic events.
The concept of "fragility curves," which represent the probability of various levels of damage in relation to specific ground motion intensity measures, is becoming increasingly integral to the design process. This tool helps engineers quantify risk and inform the development of adaptive isolation systems.
A final interesting trend is the growing focus on post-event structural recoverability. We're recognizing that systems need to be designed not only to mitigate seismic impact but also to facilitate rapid restoration efforts. This emphasis on post-event resilience underscores the importance of incorporating recovery-oriented design principles into the integration of ground motion considerations.
Revolutionize structural engineering with AI-powered analysis and design. Transform blueprints into intelligent solutions in minutes. (Get started for free)
More Posts from aistructuralreview.com: