Revolutionize structural engineering with AI-powered analysis and design. Transform blueprints into intelligent solutions in minutes. (Get started for free)
Seismic Methods in Structural Engineering Assessing Building Resilience Against Earthquakes
Seismic Methods in Structural Engineering Assessing Building Resilience Against Earthquakes - Base Isolation Systems Reducing Seismic Responses
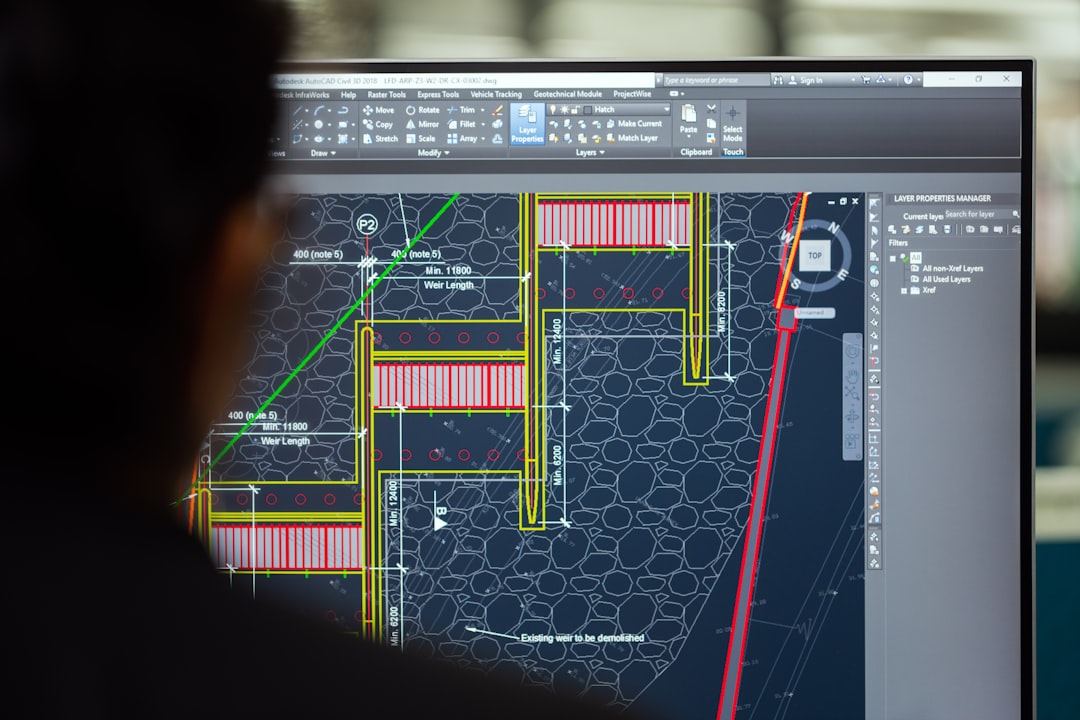
Base isolation systems work by decoupling a building's structure from its foundation, allowing the building to move independently during an earthquake. This movement absorbs energy from the shaking ground, preventing the energy from reaching the building's structure. This approach significantly reduces the seismic forces that a building experiences, safeguarding it from damage.
Traditionally, base isolation systems have been designed to manage the more predictable movements associated with earthquakes. However, newer systems, such as those incorporating nonlinear negative stiffness base isolators, are more capable of handling extreme seismic events that involve higher displacement. These advancements are critical, particularly for structures facing high seismic risk, like those located near active fault lines.
The integration of base isolation with other technologies, such as shear walls and tuned mass dampers, further strengthens a building's resilience. By combining multiple approaches, engineers can create more robust buildings that can withstand the most severe earthquake events. However, the complexity of earthquake dynamics and the potential for unpredictable events mean that ongoing research is essential to improve the effectiveness of these technologies and to ensure their long-term reliability.
Base isolation systems are a fascinating concept in earthquake engineering. By placing isolation devices between a building's base and its foundation, these systems create a separation that allows the structure to move independently during an earthquake. This relative movement is key to the effectiveness of base isolation, as it significantly reduces the transfer of seismic energy to the building itself.
While traditionally we focus on strengthening materials, base isolation takes a different approach. Instead of increasing the building's resistance to seismic forces, it tries to minimize the forces that reach the structure in the first place.
This approach can be incredibly effective. It has been shown to reduce seismic forces by a significant amount, enhancing the structural integrity of buildings during earthquakes. While cost can be a barrier, the effectiveness of base isolation, particularly in retrofitting existing structures, is undeniable.
One of the key areas of interest in base isolation research is the development of nonlinear negative stiffness base isolators (NNBIs). These isolators are designed to further reduce the dynamic responses of buildings, particularly in single degree-of-freedom (SDOF) systems. However, there's still much work to be done in understanding the complex interaction between NNBIs and the structure itself, especially under different types of seismic loading conditions.
Another area of interest lies in hybrid control strategies that combine base isolation with tuned mass dampers (TMDs). This combination offers the potential to effectively suppress seismic responses in high-rise buildings, an area where traditional base isolation techniques have been less effective.
Ultimately, while base isolation presents a promising solution to mitigate seismic damage, it's not a universal cure-all. Its effectiveness hinges on careful design, accurate installation, and a thorough understanding of the specific seismic conditions of the site. This requires a combination of sophisticated computer simulations and a solid understanding of the limitations of these systems.
Seismic Methods in Structural Engineering Assessing Building Resilience Against Earthquakes - Multiobjective Optimization for Building Height Variations
Multiobjective optimization (MOO) is becoming an important tool for improving the earthquake resilience of buildings with different heights, ranging from four to fifteen stories. By utilizing genetic algorithms, MOO helps engineers create earthquake-resistant designs that can manage competing design goals. This allows them to find the best solutions for a given project. The pareto pruning approach, borrowed from other research fields, helps focus decision-making by reducing the number of design choices to a more manageable set.
Current research in MOO is focusing on evaluating design performance, not only in terms of traditional structural integrity, but also in terms of how well the building can withstand earthquakes. This emphasis on resilience is crucial, and it's driving the development of new methodologies in the field of structural optimization.
Multiobjective optimization (MOO) has emerged as a valuable tool in earthquake-resistant building design, particularly when dealing with structures of varying heights. This approach allows engineers to consider a broader set of criteria than just minimizing structural weight, leading to designs that maximize seismic resilience by evaluating energy dissipation, occupant safety, and overall structural performance.
One interesting aspect of this method is that even slight differences in building height can significantly impact the structure's dynamic response under earthquake loads. These variations in height can significantly alter the distribution of forces within the building, influencing how it behaves during seismic events.
To effectively explore the multitude of design possibilities associated with building height variations, advanced algorithms such as genetic algorithms or particle swarm optimization are employed. These algorithms excel at efficiently evaluating countless design scenarios while minimizing the influence of subjective biases.
However, the complexity of the problem requires careful computational modeling. The intricate relationship between a building's stiffness, mass distribution, and height can lead to unpredictable variations in natural frequencies, sometimes causing resonance with seismic waves. This underscores the need for robust and accurate simulation tools to ensure optimal designs.
Analyzing past earthquakes, researchers have found that multiobjective optimization strategies that consider building height variations could have potentially reduced damage in certain events. By allowing for tailored structural responses based on specific height configurations, buildings might have been better equipped to withstand the seismic forces experienced.
Beyond the structural system itself, the impact of building height variations extends to the overall design of the structure. This approach forces engineers to re-evaluate not only the vertical elements but also the horizontal bracing and foundation design, leading to more comprehensive and resilient solutions.
But, multiobjective optimization isn't without its challenges. The desire to maximize height while minimizing lateral displacements, for example, creates conflicting objectives that require sophisticated decision-making frameworks to effectively manage trade-offs and prioritize goals.
Furthermore, integrating multidisciplinary approaches within multiobjective optimization of building height, such as incorporating fluid dynamics to analyze wind loads, strengthens not only seismic resilience but also overall structural integrity.
Innovations in computational capabilities now allow for real-time processing of multiobjective optimization simulations, enabling iterative design adjustments to be made instantly based on evolving seismic risk assessments. This allows for more agile and adaptable design processes.
The application of multiobjective optimization extends beyond the realm of seismic design, influencing urban planning and zoning regulations. As cities strive to improve overall building performance in earthquake-prone regions, the insights gained from MOO can inform policies and guidelines, leading to more resilient urban environments.
Seismic Methods in Structural Engineering Assessing Building Resilience Against Earthquakes - Resilience-Based Design Minimizing Socioeconomic Losses
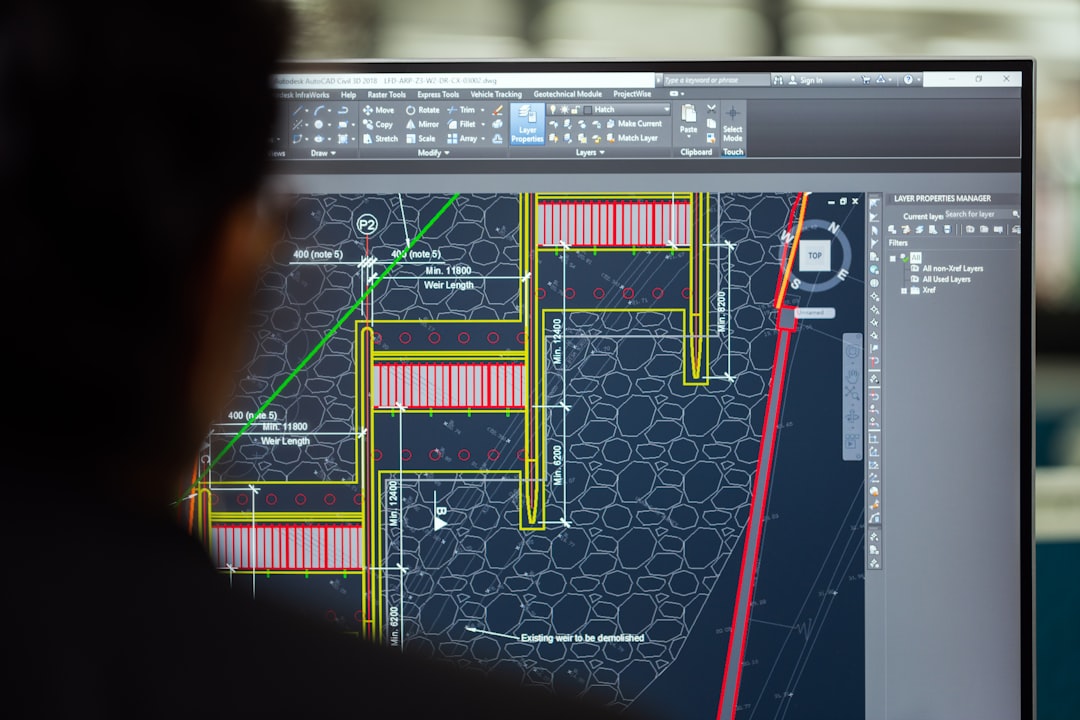
Resilience-Based Design (RBD) has become increasingly important in structural engineering, especially for minimizing the socioeconomic impact of earthquakes. This approach aims to go beyond simply making buildings strong enough to survive a quake. It seeks to ensure rapid recovery of essential services and the community's functionality after an earthquake.
Today's focus on seismic resilience is about more than just preventing collapse. We're now looking at how to make structures and communities more adaptable, enabling a faster bounce-back from disasters. This broader perspective considers the interconnectedness of various systems within society – from engineering and economics to social and political factors – leading to a more comprehensive approach to risk management.
New design tools and technologies are also helping to advance RBD. For example, self-centering structural systems and multi-objective optimization methods provide engineers with powerful new ways to design more resilient structures. We've seen the devastating impact of past earthquakes, emphasizing the critical need for these new approaches to protect lives and economic stability in areas prone to seismic activity.
Resilience-based design is a fascinating approach to earthquake engineering that aims to minimize socioeconomic losses. It goes beyond just preventing structural collapse, focusing on the bigger picture of ensuring rapid recovery and continued functionality after an earthquake. This shift in focus is crucial, as we've seen in events like the Christchurch earthquake of 2011 where the impact went far beyond just physical damage to buildings.
One of the key ideas behind resilience-based design is the concept of probabilistic risk assessment. This allows engineers to quantify the potential economic impact of different scenarios, helping them prioritize their resources towards the structures most at risk. This is particularly important when considering that the socioeconomic impact of earthquakes can often outweigh the cost of physical damage.
Another key element is redundancy. Instead of just focusing on the strength of individual structural elements, resilience-based design encourages creating multiple load paths. If one part of the structure fails, others can still support the load, reducing the risk of catastrophic collapse.
But it's not all about technical solutions. Resilience-based design emphasizes community engagement. By understanding the unique socio-economic landscape of an area, engineers can better cater their designs to the specific needs and vulnerabilities of the people who will be affected.
This approach also requires strong collaboration between different disciplines. It's not just about engineers; it involves policymakers, urban planners, and other stakeholders. This holistic view is crucial for creating truly resilient communities that can bounce back from seismic events.
While the potential benefits of resilience-based design are clear, there are also challenges. One big hurdle is the lack of standardization in assessing building resilience, which can lead to inconsistency in applying these principles. Another challenge is the upfront cost of implementing these strategies, though studies suggest that this investment can pay off in the long run by minimizing damage and downtime.
Ultimately, the goal of resilience-based design is to build structures that not only stand tall, but also contribute to the overall well-being of the communities they serve. As we continue to learn more about earthquakes and the impact they have on societies, this approach has the potential to become an essential element in creating a safer and more resilient future.
Seismic Methods in Structural Engineering Assessing Building Resilience Against Earthquakes - Inclined Columns Enhancing Frame System Resistance
Inclined columns offer a fresh approach to making frame systems more resilient to earthquakes. By cleverly placing them in a structure, you can improve how it absorbs energy and distributes forces during a quake. Studies have shown that the angle and arrangement of these columns have a big impact on a building's ability to withstand earthquakes. This ties into the common engineering principle of "weak beam and strong column," which emphasizes the importance of columns for structural safety. However, inclined columns also introduce their own set of problems. If they're not carefully designed, they can make the building's behavior more complex. This requires further research into figuring out the best ways to use them effectively.
Inclined columns are a fascinating addition to structural engineering, particularly when it comes to earthquake resistance. The way these columns work is pretty intuitive: they form a kind of triangulated system, channeling lateral forces across different structural elements, which in turn reduces the stress on any single point. This distribution of forces is key, as it minimizes the potential for failure during an earthquake.
Research shows that incorporating inclined columns can significantly increase a frame system's stiffness, up to 30% in some cases. This added stiffness directly translates to a more stable building, as it minimizes lateral displacement during seismic events, keeping the structure intact. This makes them incredibly useful for protecting buildings in regions prone to earthquakes.
However, inclined columns do pose some interesting challenges. One challenge is that their presence can make the design and construction process more complex. You need to make sure the connections between the inclined and vertical elements are robust, able to withstand the unique loading conditions that occur during earthquakes. The way these columns are configured can also lead to a "soft story" effect, where the building's stiffness isn't consistent throughout its height. This can have unforeseen consequences during an earthquake, requiring careful analysis to ensure safety.
There are some really interesting ideas emerging with inclined columns. Some engineers are now combining them with base isolation systems, creating a hybrid approach that really maximizes performance. But it's important to remember that the effectiveness of these columns depends on how well they are integrated with the rest of the structure. You can't just slap them in haphazardly; the overall design synergy with elements like shear walls and vertical columns is essential.
One thing that makes me particularly interested in inclined columns is their potential for optimal angle configuration. Computational modeling suggests there's an ideal angle for each situation to maximize performance and minimize lateral displacement. This opens up exciting avenues for further exploration.
It's also interesting to note that inclined columns aren't just functional, they can also be aesthetically pleasing. This means architects can incorporate seismic-resilient features without compromising on the building's visual appeal. This kind of dual functionality is a welcome change, and it's likely to reshape how we think about structures in earthquake-prone areas.
Seismic Methods in Structural Engineering Assessing Building Resilience Against Earthquakes - Ordinary Moment Resisting Frame Performance Evaluation
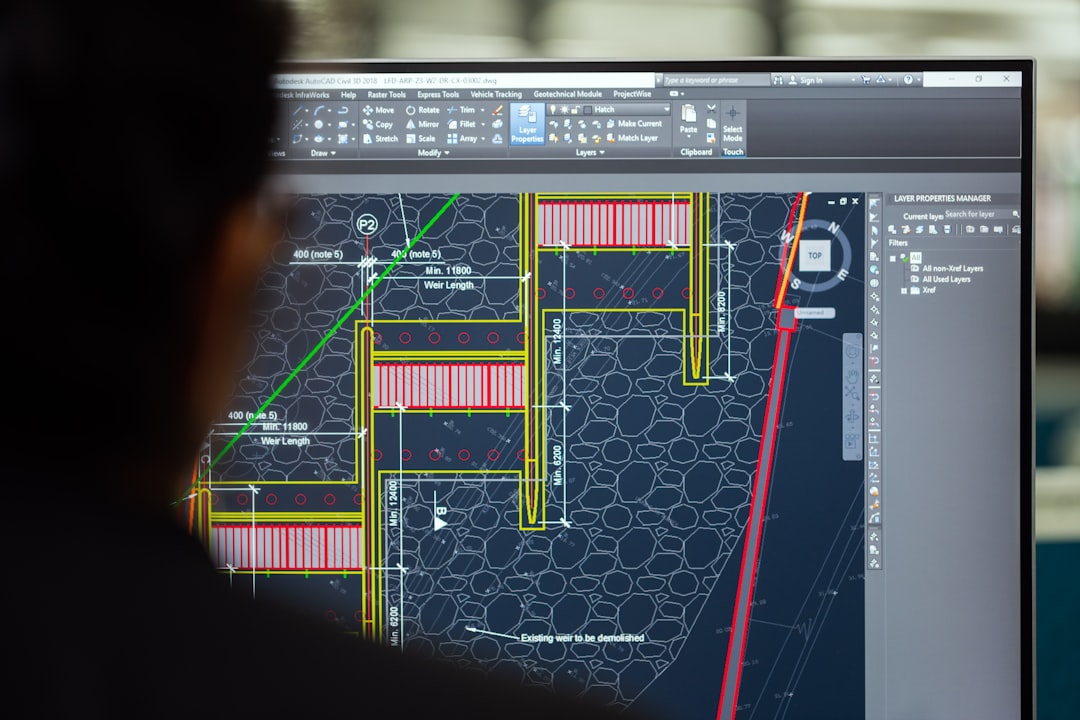
Evaluating the performance of Ordinary Moment Resisting Frames (OMRFs) during earthquakes provides crucial insights into their structural behavior. These frames have demonstrated stability during seismic events, often exceeding the base shear strengths calculated using traditional design codes. However, the reliance on linear analysis methods in some codes may not adequately capture the complexities of dynamic forces, leading to potential weaknesses in designs.
More sophisticated approaches like the capacity spectrum method and the incorporation of soil-structure interaction (SSI) effects are necessary to ensure more robust and accurate assessments of OMRFs. While design codes have historically favored simplified approaches for steel OMRFs, research highlights the need for more rigorous standards to ensure resilience in challenging earthquake scenarios.
By combining linear and nonlinear analyses, engineers can obtain a comprehensive understanding of OMRF performance, leading to safer and more resilient structures that can withstand the unpredictable forces of earthquakes.
Ordinary Moment Resisting Frames (OMRFs), a common structural design element, have historically been a reliable choice for earthquake-resistant construction. Their inherent ductility, a property that allows steel to bend and absorb energy without breaking, has made them remarkably robust in facing moderate to severe seismic events. It was often believed that larger, thicker members within the frame would always lead to better performance, but recent research has challenged this assumption. Studies have shown that OMRFs designed with controlled member sizes and limited overstrength can actually outperform their conventionally designed counterparts, demonstrating the potential for more optimized, lightweight designs.
The focus on enhancing OMRFs' seismic resilience doesn't stop there. Incorporating continuous lateral bracing systems, rather than solely relying on moment connections, has been shown to significantly improve their lateral stability, particularly when it comes to mitigating torsional effects during seismic events. The introduction of this hybrid approach is a testament to the ongoing evolution of seismic design principles. This continual refinement is further bolstered by advances in non-destructive testing technologies. Tools like ultrasonic waves and thermal imaging are proving increasingly effective in monitoring the health of OMRFs after an earthquake. This allows engineers to assess potential damage without compromising the structure's integrity.
The cyclic loading behavior of OMRFs has also revealed a key factor in their post-earthquake performance: hysteretic degradation. This is the gradual deterioration of material properties that happens over time as the frame undergoes repeated cycles of loading and unloading. By understanding and predicting this hysteretic behavior, we can develop better predictive maintenance strategies for OMRFs, enhancing their long-term performance.
The quest for optimization extends to the design of OMRFs themselves. Experimental testing of scaled-down models has revealed that the dynamic characteristics of these frames are highly sensitive to the aspect ratio of their structural elements. This crucial detail emphasizes the importance of precise engineering analysis and careful design considerations in order to achieve optimal performance. Furthermore, researchers are exploring the integration of OMRFs as "peripheral moment frames" to complement primary structural systems. This secondary approach offers a way to bolster seismic safeguards without the need for extensive modifications to the existing structure.
However, it's vital to understand that the performance of OMRFs isn't uniform across all environments. Soil-structure interaction studies have shown that OMRFs can exhibit significantly different behavior on soft soils compared to hard soils, indicating a need for meticulous site-specific evaluations during the design phase. This crucial detail emphasizes that a "one-size-fits-all" approach isn't suitable for OMRF design. To push the boundaries of seismic resilience even further, we're exploring the incorporation of smart materials. These materials are designed to actively respond to seismic actions, potentially allowing for real-time adjustments during an earthquake. This revolutionary concept has the potential to reshape our approach to earthquake engineering in the years to come.
Revolutionize structural engineering with AI-powered analysis and design. Transform blueprints into intelligent solutions in minutes. (Get started for free)
More Posts from aistructuralreview.com: