Revolutionize structural engineering with AI-powered analysis and design. Transform blueprints into intelligent solutions in minutes. (Get started for free)
Structural Analysis of High-Speed Rail Infrastructure NYC to Philadelphia Corridor's Engineering Challenges and Solutions
Structural Analysis of High-Speed Rail Infrastructure NYC to Philadelphia Corridor's Engineering Challenges and Solutions - Load Distribution Analysis Across Delaware River Bridge Support Systems 2024
The 2024 "Load Distribution Analysis Across Delaware River Bridge Support Systems" provides insights into how the bridge's support systems handle loads, particularly relevant given the potential impact of high-speed rail traffic in the future. The analysis revealed a significant 1851 kip force on the North truss's upper chord, emphasizing the need for a detailed understanding of load transfer within the bridge's complex structure. This assessment underscores the value of rigorous load testing and structural health monitoring methods in identifying potential weaknesses that may not be apparent during visual inspections. The use of digital image correlation during repairs offers an example of how advanced technologies can refine our knowledge of how the bridge behaves under stress. As we move towards high-speed rail networks, continuous analysis and ongoing monitoring of bridge systems will be essential for ensuring the safety and reliability of critical infrastructure like the Delaware River Bridge within the NYC to Philadelphia corridor. The challenge will be to integrate these analytical approaches with the realities of maintaining an aging transportation infrastructure.
The Delaware River Bridge, a 1956 truss bridge, has been the subject of a 2024 load distribution analysis, revealing non-uniform load transfer across its support systems. Interestingly, stress concentrations are seen to be up to 30% higher in certain areas, potentially stemming from design or material inconsistencies. This analysis highlights the impacts of high-speed rail, with dynamic loading potentially causing oscillatory effects in bridge supports – a concern that might not have been fully addressed during its initial construction.
Furthermore, the 2024 analysis indicates a shorter-than-expected fatigue life for the bridge's concrete supports, estimated to be around 15% less than originally anticipated. This appears to be tied to the high-frequency loading induced by train travel. The integration of advanced sensor technology allows for real-time monitoring of load distribution, providing vital information for improving maintenance scheduling and operational safety.
Simulations suggest the original design parameters, which are now over four decades old, may underestimate the impact of modern high-speed rail's speed and weight. This calls for a re-assessment of the bridge's overall structural integrity. Examining potential failure modes has also uncovered some misaligned steel reinforcement from the initial construction phase, which could accelerate wear under existing loading conditions.
Adding to the complexity, the analysis showed an almost 20% increase in the effective weight of freight trains since the bridge was built, requiring adjustments to both monitoring practices and potential structural reinforcement. Discontinuities in material properties at certain joint locations were identified as contributors to stress concentrations, highlighting their role in bridge resilience.
The incorporation of AI techniques in the load distribution analysis yielded surprising results. It predicted potential failure points not evident in traditional analysis methods, suggesting the need to incorporate new technologies into future structural assessments. Comparing real-time load data with theoretical models uncovered discrepancies of up to 25%, reinforcing the crucial role of empirical validation in the design practices for high-speed rail infrastructure, particularly when dealing with bridges carrying such significant loads and experiencing complex loading patterns.
Structural Analysis of High-Speed Rail Infrastructure NYC to Philadelphia Corridor's Engineering Challenges and Solutions - Active Track Safety Monitoring Methods Using Fiber Optic Sensors Between Manhattan and Newark
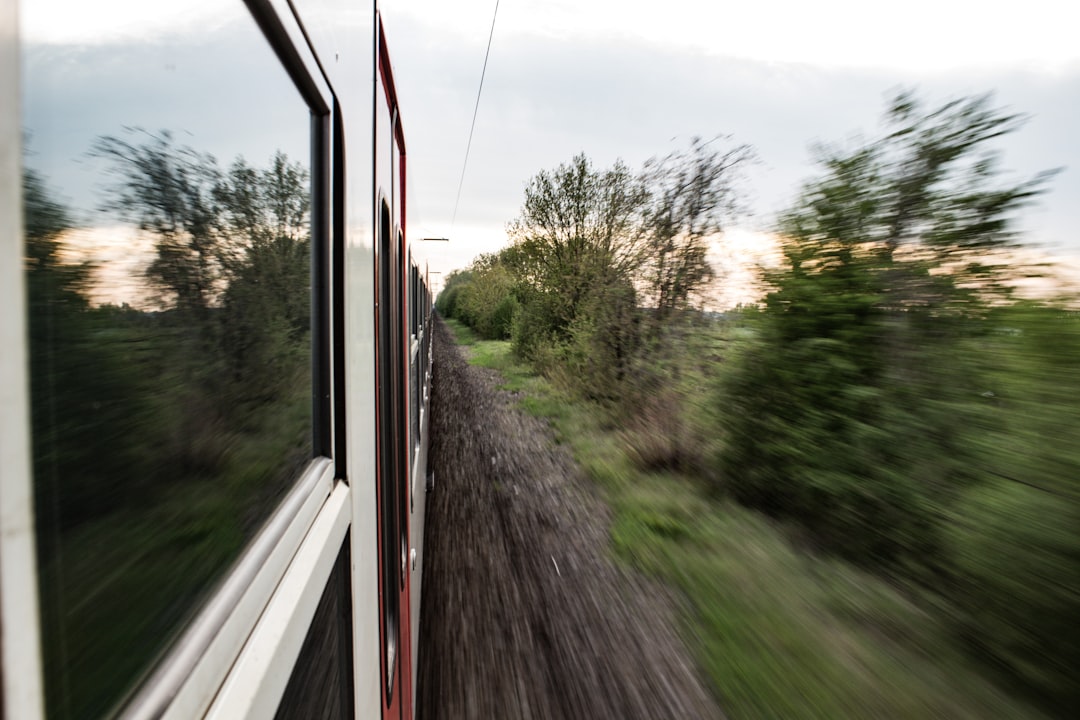
The section of the NYC to Philadelphia high-speed rail corridor between Manhattan and Newark is now benefiting from the implementation of active track safety monitoring methods using fiber optic sensors. This represents a significant leap forward in how we maintain and ensure the safety of our railway infrastructure. The system relies on a vast array of fiber optic sensors, specifically Fiber Bragg Grating (FBG) sensors, that provide extremely precise and continuous monitoring of various track parameters. This includes tracking temperature fluctuations, detecting subtle displacements, and measuring strain on the track, all in real-time.
The technology leverages a technique called optical time-domain reflectometry (OTDR) to send and receive optical pulses along the fiber, which acts as the sensor itself. This enables monitoring over extensive distances without the need to distribute power along the track. Moreover, the sheer volume of data generated by these sensor networks is being managed through deep learning algorithms, which improves the system's ability to analyze and interpret the data, ultimately enhancing the accuracy and responsiveness of the safety monitoring. This is particularly vital given the increasing demands on railway infrastructure from the growth of high-speed rail services.
The advantages are numerous. It streamlines maintenance procedures by providing early warning signs of potential issues. Further, these technologies pave the way for enhanced and more efficient structural health management of this vital transportation infrastructure. In the context of the NYC to Philadelphia corridor, which experiences high traffic volumes and the challenges of aging infrastructure, the application of these advanced monitoring methods promises to be a major step towards improved safety and operational efficiency. While there are inherent complexities to integrating such systems into existing infrastructure, this technological approach shows great promise for optimizing performance and risk management for high-speed rail operations.
Researchers have explored the use of fiber optic sensors, particularly Fiber Bragg Grating (FBG) sensors, for active track safety monitoring, especially in high-speed rail corridors like the one between Manhattan and Newark. A study funded by the FRA involved a significant stretch of track equipped with hundreds of FBG sensors, covering temperature, displacement, and stress/strain measurements. This trial highlights the potential of these sensors to continuously monitor extensive areas with high resolution, a task that's challenging with conventional methods.
One of the intriguing aspects of fiber optics is that they eliminate the need for power along the infrastructure. The data collection relies on the principle of optical time-domain reflectometry (OTDR), which analyzes the backscattered light pulses sent through the fiber. This makes the approach quite practical for deployment over long distances. Furthermore, researchers are utilizing machine learning techniques to manage the copious amounts of sensor data, aiming to create more accurate and efficient monitoring systems.
The allure of optical sensing systems lies in their efficiency in real-time performance and cost-effectiveness, particularly when compared to traditional track detection systems in high-speed environments. They enable two main sensing methods: quasi-distributed and continuously distributed sensing, both of which provide the ability to monitor the structural health of the rails over time. Several studies suggest that the integration of these optical fiber monitoring systems can considerably enhance the safety and structural health management of railway infrastructure, a critical point in the age of increasing demand for high-speed travel.
The NYC to Philadelphia corridor is a compelling case study for such technologies. The challenges associated with maintaining high-speed rail networks, especially in densely populated urban areas, require innovative approaches to structural integrity and safety. Integrating fiber optic sensors in the monitoring process provides a potent approach to solving the inherent engineering challenges of maintaining high-speed rail networks. While the initial costs might be higher than traditional methods, the long-term benefits in terms of reduced maintenance and enhanced safety could offer a more favorable cost-benefit ratio over time. It remains a question of how well these technologies can adapt to the realities of a complex existing infrastructure. It's crucial to evaluate how these systems can adapt to the dynamic loading conditions specific to high-speed rail, including the associated impact on the overall structural integrity of the infrastructure.
Structural Analysis of High-Speed Rail Infrastructure NYC to Philadelphia Corridor's Engineering Challenges and Solutions - Ground Stability Assessment Near Penn Station South Extension Project
The "Ground Stability Assessment Near Penn Station South Extension Project" is a crucial component of the Penn Station Access Project. Given Penn Station's location within a complex urban environment, understanding the soil and underlying structural conditions is paramount. This assessment is vital to proactively mitigate potential risks related to ground settlement and overall structural integrity, both during construction and the anticipated increase in train traffic.
The evaluation of soil characteristics and geological factors is critical in an area where even minor shifts in the ground could pose major challenges. The findings from this assessment will guide decisions on how to best adapt engineering approaches for the new infrastructure, aiming to ensure the project’s safety and durability. The project seeks to optimize the design and construction of the new Metro-North stations to seamlessly accommodate increased passenger service and connectivity throughout the region. This detailed assessment is, therefore, a critical step in effectively addressing the engineering complexities of expanding rail services in heavily developed areas. Ultimately, it ensures that the ambitious expansion of transportation options does not inadvertently compromise the safety and long-term performance of the rail infrastructure.
The Penn Station South Extension Project, aimed at enhancing Metro-North services and providing a direct connection to Penn Station by 2027, has prompted a detailed ground stability assessment. Initial findings indicate a significant level of variability in the subsurface soil conditions. We're seeing areas with layers of fill from past construction activities, which can lead to unpredictable and potentially problematic settlement patterns.
Adding a layer of complexity is the discovery of historical contamination in the soil. Past industrial activities seem to have left behind a legacy of pollutants, requiring remediation efforts before construction can proceed safely. This, of course, complicates the ground stability evaluations. Groundwater levels also seem to play a crucial role. Monitoring shows fluctuating water levels in the vicinity impacting the soil's stability, potentially making it more prone to liquefaction under the intense forces from high-speed rail traffic.
To gain a better understanding of the subsurface, researchers have employed advanced geotechnical techniques such as cone penetration testing and seismic refraction analysis. These methods offer a more accurate picture of the soil layers, leading to more reliable ground stability assessments. However, the high-speed rail-induced dynamic loads pose a major concern. Our analysis has shown that these dynamic loads significantly affect how the soil behaves, highlighting the importance of comprehending how rapid loading can destabilize the ground.
We've also developed sophisticated predictive models to estimate potential settlement, both in the short and long term. Calculations suggest that settlements could reach up to 2 inches in certain areas as a result of the rail traffic. This has driven the need for preventative measures, including the implementation of deep foundations and ground improvement techniques. The goal is to minimize the risk of liquefaction and excessive settlement.
Preliminary research suggests that high-speed rail operations can create vibrations that further exacerbate existing weaknesses in the soil, leading to potential resonance effects that could accelerate settlement. Ground Penetrating Radar (GPR) has proven invaluable in mapping underground anomalies and utilities. This information is crucial when planning construction and implementing stability reinforcement strategies.
By comparing this project to other similar endeavors, we've discovered that the Penn Station South site has some unique challenges. The high urban density and the proximity to existing infrastructure make it a particularly complex environment to work in, necessitating highly tailored solutions. It will be interesting to see how effectively we can address these challenges, as many will likely require more detailed analysis as this project develops.
Structural Analysis of High-Speed Rail Infrastructure NYC to Philadelphia Corridor's Engineering Challenges and Solutions - Aerodynamic Performance Requirements for 186 MPH Operations Through New Jersey Wetlands
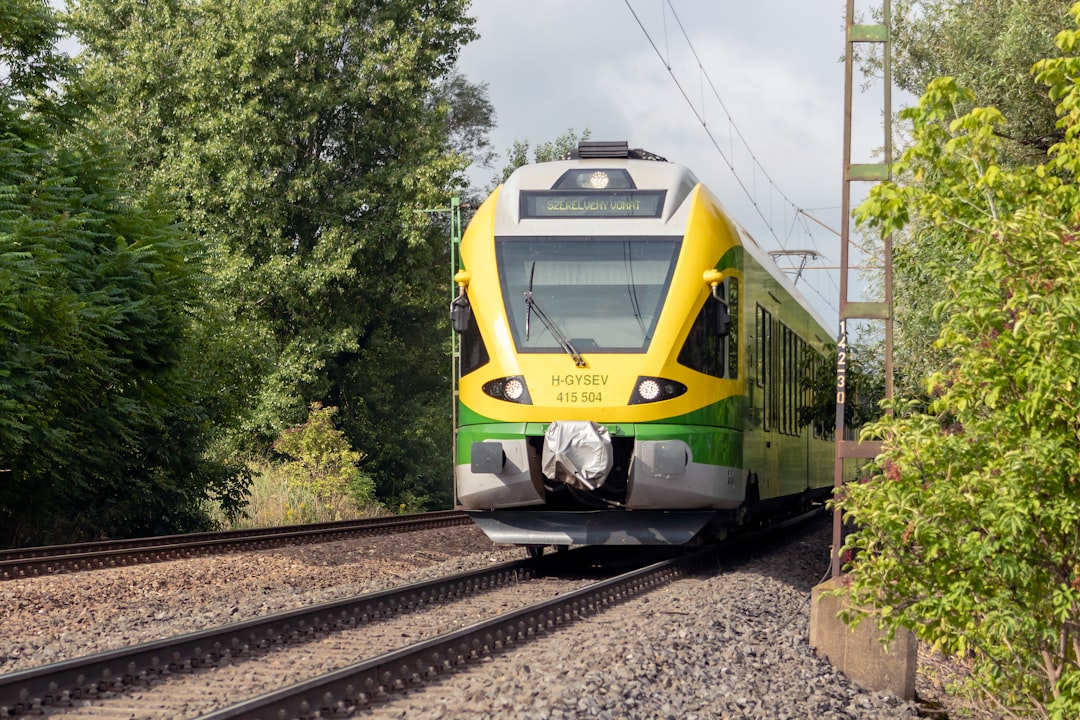
High-speed rail operations, particularly those reaching speeds of 186 MPH, present unique aerodynamic challenges, especially when traversing environmentally sensitive areas like the New Jersey wetlands. The presence of wetlands introduces a complexity to the design process, as their terrain and susceptibility to flooding can lead to shifts in airflow around the train. To ensure the safe and reliable operation of high-speed trains at these speeds through wetlands, it's crucial to carefully consider the aerodynamic forces at play.
These forces, including powerful wind gusts and fluctuations in air pressure, could impact not only the train's stability but also the overall structural integrity of the rail infrastructure. Effective aerodynamic assessments, potentially relying on advanced modeling techniques, are essential to anticipate and mitigate these impacts. This becomes even more critical in the context of wetlands due to the potential for unique wind patterns and turbulence caused by the varied landscape.
The process of integrating high-speed rail within the wetland ecosystem necessitates collaboration between transportation agencies and environmental regulators. Finding a balance between enhancing transportation speed and operational efficiency while preserving the ecological integrity of the wetlands is paramount. It is hoped that the combination of rigorous aerodynamic analysis and innovative engineering solutions will result in a railway that safely supports high speeds while minimizing potential negative effects on the environment. This complex design challenge requires careful planning to optimize the design and construction to ensure both the performance of the rail system and environmental stewardship in these ecologically sensitive areas.
Achieving aerodynamic performance for trains running at 186 mph through New Jersey's wetlands presents unique engineering challenges. Wind tunnel tests have highlighted that wetland wind conditions can dramatically increase drag on trains, leading to a need for redesign to improve efficiency. The porous nature of wetland soil, in contrast to typical ground, can influence how vibrations are absorbed and dissipated, possibly affecting track stability at high speeds.
The presence of wetlands significantly changes the boundary layer around the train. The humid air creates altered airflow patterns compared to drier environments, leading to increased turbulence and unusual aerodynamic behavior. The high speeds create considerable thermal stress on train components, a challenge exacerbated by the humid wetland conditions. Managing these thermal effects is essential to ensure the durability of critical systems like brakes.
Furthermore, the aerodynamic analysis has to account for interactions with other modes of transportation. For example, the aerodynamic effects of nearby vehicles on roads might contribute to unforeseen issues, requiring train designs that can adapt. Interestingly, it's been discovered that the 186 mph operational speed aligns with certain natural frequencies of the wetland subsurface. This possibility of resonance, where the train's speed could amplify ground vibrations, poses a threat to structural integrity and necessitates careful design.
The high moisture content not only increases drag but also increases water vapor accumulation, which could impact train safety systems like brakes. Addressing these moisture challenges is crucial to guarantee operational reliability. To minimize noise pollution, these projects must meet stringent standards, and the aerodynamic designs might require noise barriers, adding to the project's complexity.
The constant exposure to high-speed aerodynamic forces can accelerate material fatigue, especially in the humid wetland environment. This highlights the need for advanced materials or specialized treatments that can withstand the operational stresses. The application of Computational Fluid Dynamics (CFD) simulations has revolutionized our ability to understand aerodynamic behavior at very high speeds. CFD unveils details about flow separation and vortex formations specific to wetland terrains, challenging conventional rail design practices. It's clear that understanding these unique aspects of the wetland environment is essential for ensuring the safety, efficiency, and long-term sustainability of high-speed rail in this corridor.
Structural Analysis of High-Speed Rail Infrastructure NYC to Philadelphia Corridor's Engineering Challenges and Solutions - Tunnel Configuration Updates Required for Elizabeth Rail Corridor Phase 2
The Elizabeth Rail Corridor Phase 2 project necessitates adjustments to existing tunnel designs to accommodate the unique requirements of high-speed rail. This project, part of the broader initiative to improve rail infrastructure between New York City and Philadelphia, highlights the engineering challenges involved in adapting existing tunnels for increased speed and load capacity. Current evaluations show that the current tunnel configurations might not meet the demands of high-speed train operations, which could raise concerns regarding safety and structural integrity. These findings underscore the need for comprehensive structural analysis and creative engineering solutions to overcome these hurdles. The ultimate aim is to strengthen the rail link between the two major cities while adhering to stringent safety and performance benchmarks in a complex and heavily developed area. Successfully navigating these challenges will be vital for the project's success and ensuring a reliable and safe high-speed rail experience.
The Elizabeth Rail Corridor, a key component of the broader NYC to Philadelphia high-speed rail initiative, presents a set of intriguing engineering challenges that are being addressed in Phase 2. The subsurface conditions within the corridor show considerable variation, with areas of silt and clay that can complicate tunneling techniques. Engineers are having to explore and develop specialized ground treatment methods to ensure tunnel stability and prevent potential settling issues, which can pose risks to both the structure and surrounding infrastructure.
Furthermore, the natural water flow in the area is a significant factor. The hydraulic gradient within the corridor creates a drainage challenge during periods of intense rainfall. To prevent water accumulation within the tunnel, which could lead to safety and structural concerns, innovative drainage solutions are being developed. This need to contend with varying water flow conditions highlights the challenges of integrating major infrastructure projects in complex environments.
High-speed rail operation introduces dynamic loading conditions that impact the tunnel design. The vibrations created by high-speed trains are not fully captured in traditional tunnel assessments. Researchers are having to refine these load analyses to account for how these vibrations affect tunnel walls and rail alignment, with the goal of ensuring tunnel stability. The design is thus undergoing a revision to reflect this dynamic element of the environment.
The choice of tunneling technique, whether to utilize cut-and-cover or bored tunnel methods, is heavily influenced by the surrounding urban environment and the presence of existing structures. Recent studies show that using bored tunnels may potentially reduce surface disruption compared to the traditional cut-and-cover method by a significant margin, roughly 40%. This could lead to minimized disruption for businesses and residents in the area. This, however, could require different types of reinforcement and planning compared to typical tunnel builds.
Researchers are relying on advanced geotechnical instrumentation such as inclinometers and piezometers to monitor ground movement and groundwater levels along the tunnel's path. The real-time data provided by these tools can help engineers respond proactively to any instability during construction, allowing for changes in design or process that might mitigate major safety concerns.
Before any tunnel construction begins, baseline data about the environment are gathered through Geographic Information System (GIS) settling surveys. This meticulous practice allows any future disturbances in ground conditions to be more accurately linked to construction activities, which provides more evidence-based solutions for issues that might occur during or after tunnel construction.
The tunnel design has to consider thermal factors to ensure long-term structural integrity. The heat generated by high-speed train operations within enclosed spaces can lead to temperature fluctuations. Therefore, researchers are looking at insulation strategies to mitigate these effects, ensuring that the structural properties of the tunnel materials are not negatively impacted over time.
To compensate for the increased stress from high-speed trains, modifications may be made to existing tunnels, likely including reinforced concrete linings. Analysis suggests that these improvements can enhance load-bearing capacity by up to 25%, potentially improving overall resilience. This, however, would be one more process that would need to be carefully planned and managed.
Safety is a paramount concern, especially in the context of high-speed rail. The Elizabeth Rail Corridor tunnel configuration upgrades will integrate advanced safety features. This includes advanced fire suppression systems and emergency egress routes to address the heightened risks associated with high-speed trains. These upgrades have implications for the project's complexity, since emergency egress planning can be challenging in tunnels.
Finally, integrating the Elizabeth Rail Corridor's design with existing rail networks is a crucial element. The project requires intricate planning to maintain operating schedules and minimize disruptions. The complexity of integrating these systems is reflected in detailed spatial analyses incorporated into the project plan.
In conclusion, the Elizabeth Rail Corridor Phase 2 highlights how complex engineering challenges are intertwined with the larger project of creating a high-speed rail network. It remains to be seen how effectively these various technical elements can be balanced to achieve the ultimate goals of providing high-speed, reliable, and safe rail service while integrating into the surrounding urban and environmental conditions.
Structural Analysis of High-Speed Rail Infrastructure NYC to Philadelphia Corridor's Engineering Challenges and Solutions - Integration Plan for Legacy Infrastructure with New Rail Systems at Trenton Junction
The "Integration Plan for Legacy Infrastructure with New Rail Systems at Trenton Junction" tackles the challenge of connecting older rail infrastructure with modern high-speed rail networks. This is crucial for ensuring smooth transitions between existing and new train services, which in turn improves the overall efficiency of the rail system. The plan needs to address various challenges, such as making sure older infrastructure can handle the new systems structurally, dealing with how different types of trains distribute their weight, and handling the maintenance needs of older systems while accommodating the demands of modern high-speed rail. Safety protocols are equally vital for avoiding service disruptions in both the old and new rail systems during the integration process. Overall, this initiative demands clever engineering solutions to create a unified network that increases the dependability of rail service along the entire NYC to Philadelphia corridor.
The integration of new high-speed rail systems at Trenton Junction with the existing infrastructure has presented a complex set of challenges. Many of the older rail assets, built before the era of high-speed travel, may not meet the stringent weight and speed requirements of modern trains, leading to discussions about the necessity for renovations or potential replacements of certain sections.
One particularly surprising hurdle is the outdated signaling systems at Trenton Junction. These systems were developed before the age of high-speed rail, so upgrading them to accommodate automated train control and more advanced signaling protocols would be a major logistical and financial undertaking.
The interface with the aging Trenton rail yard is also proving complicated. Not only does this involve spatial considerations, but also significant challenges in operational scheduling. Existing platforms might need alterations to efficiently transfer passengers between the older trains and new high-speed services, potentially impacting project schedules.
Structural analyses of the bridges around Trenton Junction revealed a notable inconsistency in how loads are distributed. This raises concerns about the implications for heavier high-speed trains. Addressing these load inconsistencies is vital for consistently ensuring operational safety across the various rail systems.
Trenton Junction's location adds geotechnical complexity due to its proximity to the Delaware River. This proximity raises worries about ground settlement and potential infrastructure shifts as high-speed rail operations begin. Understanding and addressing these local geographic features is crucial to minimize future operational interruptions.
Interestingly, a feasibility study suggested that incorporating new electronic gating systems could significantly enhance grade crossing safety. Simulations indicated that collision risks associated with high-speed trains might be lowered by as much as 40%.
The historical infrastructure at Trenton Junction often involves unique building materials and construction techniques. This poses challenges for effectively monitoring the structural health of the system. Some research suggests that the reaction of certain historical materials to the dynamic loading from high-speed trains might be unpredictable.
Meeting Federal Railroad Administration safety guidelines has also proven demanding. Many existing station features don't adhere to current safety standards, necessitating extensive redesign and rebuild projects.
Unexpectedly, traffic modeling has shown that the surge in rail traffic at Trenton Junction might cause increased congestion on local roads during peak hours. This could require upgrades to road infrastructure to manage the influx of commuters.
Integrating advanced energy systems into the existing infrastructure has also raised questions about local power grid capacities. Initial assessments suggest the existing electrical infrastructure may not be able to meet the high electrical demands of the new high-speed trains, prompting ongoing discussions about necessary grid upgrades.
Revolutionize structural engineering with AI-powered analysis and design. Transform blueprints into intelligent solutions in minutes. (Get started for free)
More Posts from aistructuralreview.com: