Revolutionize structural engineering with AI-powered analysis and design. Transform blueprints into intelligent solutions in minutes. (Get started for free)
Innovative Beam-to-Wall Connection Techniques for Enhanced Seismic Performance
Innovative Beam-to-Wall Connection Techniques for Enhanced Seismic Performance - Semi-rigid beam-to-column connections for steel shear walls
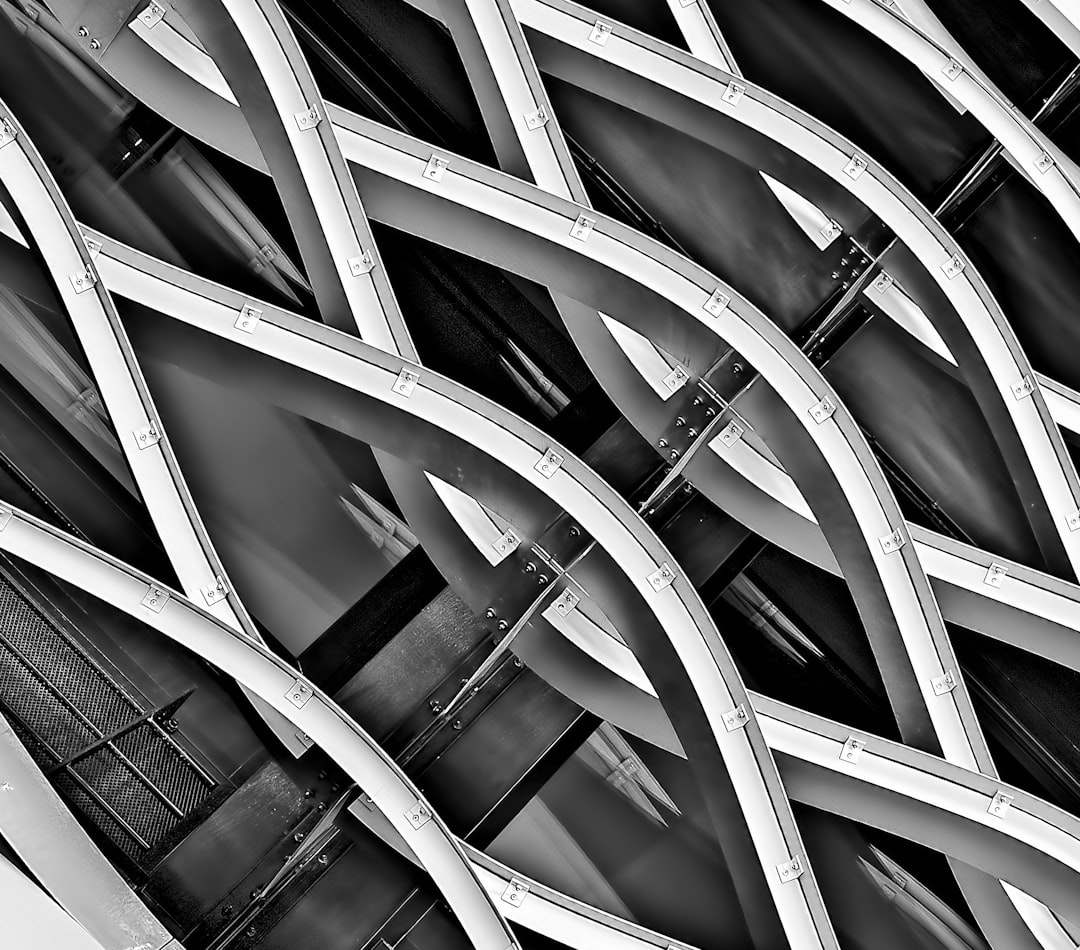
Semi-rigid beam-to-column connections are becoming increasingly popular in steel shear walls, as they offer the potential for improved seismic performance. Recent research has focused on using these connections to enhance energy dissipation during earthquakes, which is critical for mitigating damage. This approach, however, requires careful consideration of the specific stiffness characteristics of these connections, as these properties influence the overall structural behavior and resilience under dynamic loads.
While standard approaches for determining connection stiffness often rely on methods suitable for static or cyclic loads, further investigations are needed to accurately assess their performance under dynamic seismic loads. This is particularly crucial, as an understanding of these connections' performance under dynamic loads will be critical for optimizing designs that minimize plastic damage to structural elements.
Semi-rigid beam-to-column connections offer a fascinating approach to improving the seismic resilience of steel shear walls. By introducing a controlled level of rotational freedom, these connections effectively reduce stress concentrations that can arise during earthquakes, ultimately contributing to the overall ductility of the structure. This controlled flexibility, unlike traditional rigid connections, allows for a degree of displacement, enabling energy dissipation and minimizing the risk of brittle failures.
Research is showing that semi-rigid connections might even lead to more efficient use of materials. By allowing for optimized structural performance without sacrificing safety, these connections could potentially reduce the required size of beams and columns. The intriguing aspect of these connections is their ability to be engineered with variable stiffness, offering adaptability for different loading scenarios. This allows for a more nuanced response to earthquakes, potentially leading to improved building performance.
Finite element analyses have shown that semi-rigid connections can effectively distribute forces throughout the structure, minimizing localized failures and promoting a more uniform response to seismic activity. However, it's crucial to note that the performance of these connections is highly dependent on design details like bolt pretensioning and beam depths, which can significantly influence connection stiffness and the overall system behavior.
While semi-rigid connections hold great promise, their design and analysis present challenges that necessitate advanced modeling techniques for accurate prediction under varying loads. Additionally, long-term maintenance and inspection might be crucial to ensure their functionality, especially in high-seismic regions.
Despite the complexities, the potential benefits of semi-rigid connections, especially in modern, large-scale structures, make them a worthy avenue for ongoing research and development, especially as we strive to meet increasingly stringent building codes and performance-based design criteria that prioritize resilience.
Innovative Beam-to-Wall Connection Techniques for Enhanced Seismic Performance - Self-centering tension-only braces in beam-column joints
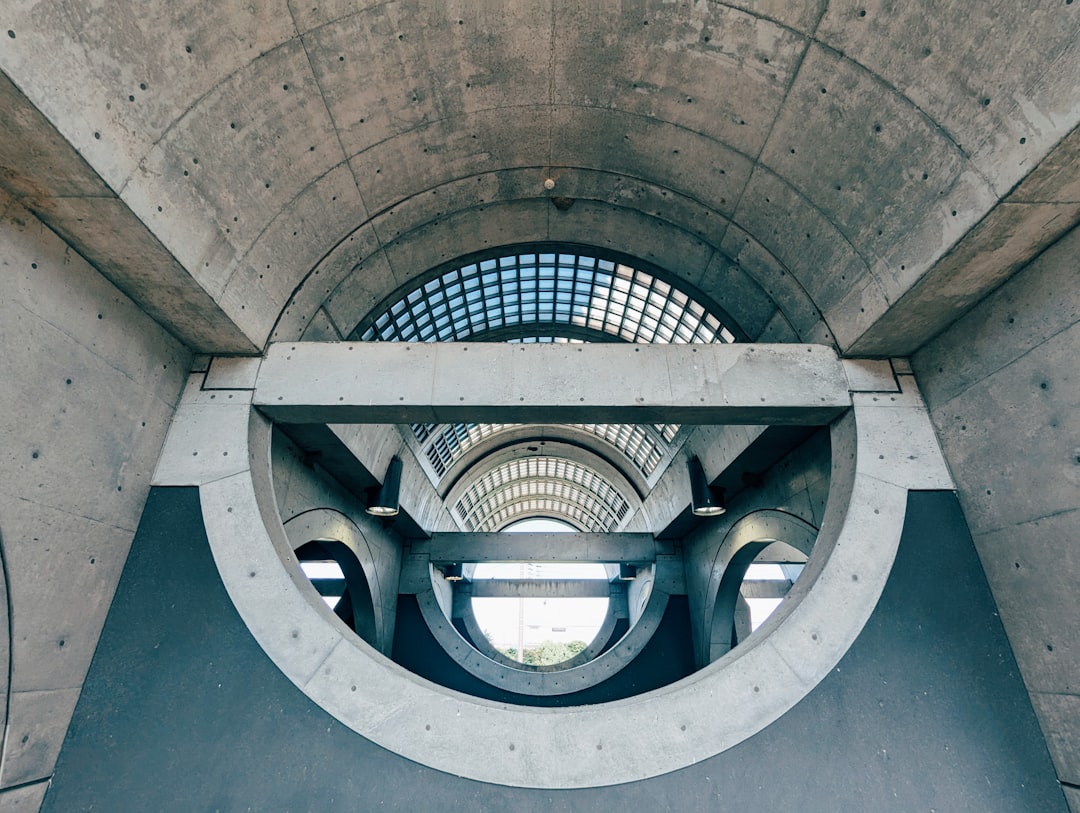
Self-centering tension-only braces (SCTOBs) are a relatively new concept in seismic design. They aim to improve the resilience of buildings by minimizing residual deformation after an earthquake. These braces incorporate self-centering elements and energy dissipation devices, working together to reduce drift and dissipate the energy generated during an earthquake.
The key innovation lies in the design of the brace ends, where a resilient slip-friction joint is introduced. This joint allows for non-pinching behavior, ensuring the brace retains its strength without degrading.
SCTOBs also benefit from initial prestress, which contributes to a uniform distribution of story drift. This is especially important in low-rise steel buildings.
While these braces show promise, more research is needed to understand their long-term performance and effectiveness in different seismic zones. The potential of SCTOBs to minimize damage and enhance structural efficiency in earthquakes makes them a worthwhile area of ongoing investigation.
Self-centering tension-only braces (SCTOBs) are gaining traction as a potentially revolutionary approach to seismic design. These braces use a clever design that allows them to "self-center" after an earthquake, returning to their original position and reducing the lingering deformation that can cause significant damage and repair costs. This self-centering action hinges on a tension-only design, meaning that the brace only experiences tensile forces, simplifying connections and potentially requiring less material compared to conventional braces.
The absence of significant compression forces also makes it possible to integrate energy dissipation devices that can absorb the energy from seismic events. This capability makes SCTOBs useful in mitigating damage from multiple hazards.
Early research is showing promising results. For instance, finite element analyses have shown that SCTOBs can lead to a reduction in integral moments in beams, which in turn minimizes bending demands and potentially extends the life of structural elements.
While SCTOBs are still relatively new, their success in real-world projects is starting to shine through. These braces are being used in structures across the globe, and their performance in reducing damage and repair costs is gaining recognition.
As an engineer, I find it exciting to see how SCTOBs are pushing the boundaries of seismic design. Their potential for improving structural resilience is undeniable, but it is crucial to conduct rigorous research and testing to fully understand their behavior under various loading scenarios. Further exploration of their long-term performance, durability, and cost-effectiveness will be essential for their broader adoption. The promise of more resilient and economical buildings makes SCTOBs a compelling avenue for continued research and development in the pursuit of safer and more sustainable structures.
Innovative Beam-to-Wall Connection Techniques for Enhanced Seismic Performance - High-performance precast concrete building connection systems
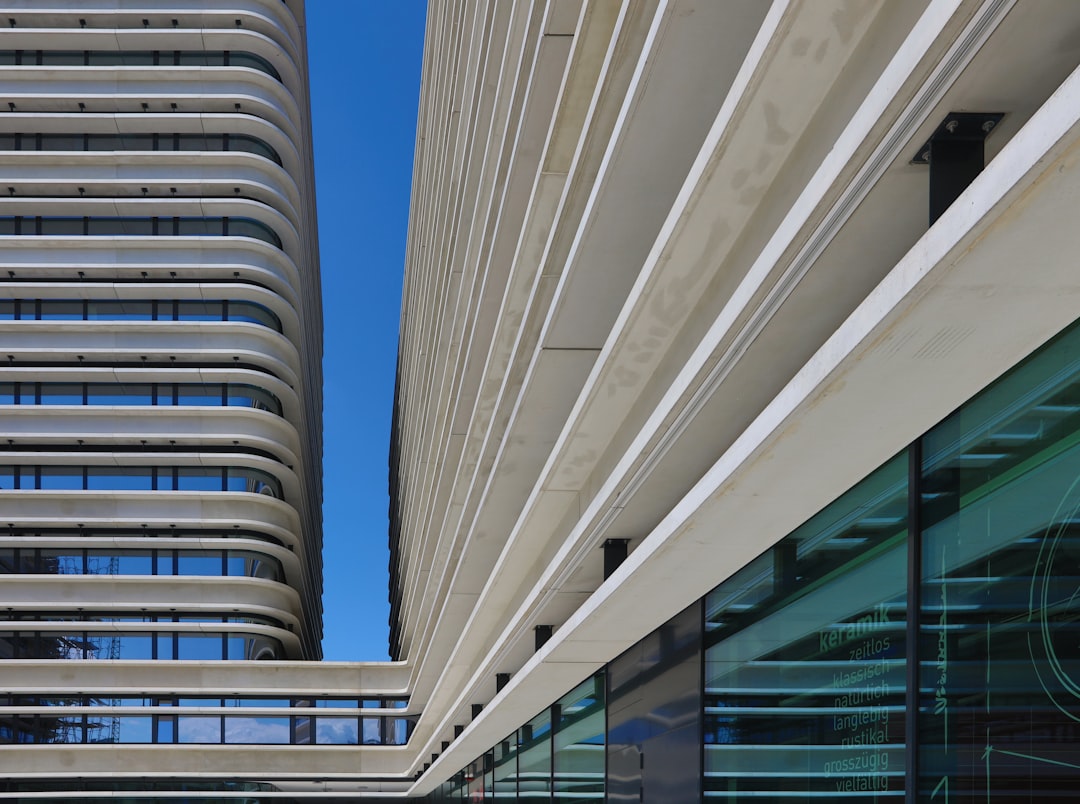
High-performance precast concrete building connection systems are a key area of development in the pursuit of structures that can withstand earthquakes. The goal of these systems is to mimic the performance of traditional, cast-in-place concrete buildings, offering increased resilience during seismic events. One promising area of exploration involves hybrid connection systems, which combine both wet and dry connections, resulting in increased energy dissipation and overall strength. The ring-lap joint is a notable example of how specific design modifications can enhance precast connection systems. It is still crucial to note that further research and development are necessary to perfect these systems, particularly with an eye towards balancing sustainability goals with ensuring the highest levels of safety during seismic events.
High-performance precast concrete building connections have emerged as a significant advancement in seismic-resistant design. These systems aim to achieve the same level of seismic resilience as traditional cast-in-place structures. One intriguing approach focuses on incorporating flexibility into these connections. Materials like rubber or advanced polymers are often used, allowing the structures to absorb earthquake energy more effectively while maintaining structural integrity during moderate seismic events.
To further enhance ductility, innovative post-tensioning techniques are employed. This process allows the connections to deform significantly without failing, a key aspect in surviving extreme seismic events. The increased ductility offered by these systems contrasts with traditional fixed connections, which are prone to catastrophic failure during strong earthquakes.
Beyond structural performance, precast connections offer logistical advantages. Their pre-fabricated nature allows for faster assembly on-site, significantly reducing labor costs and project timelines. Furthermore, many systems are designed for easy disassembly, which can benefit future renovations or rebuilding. This approach minimizes material waste and avoids extensive structural damage.
Interestingly, precast connections can lead to reduced material usage. Advanced computational modeling allows for the precise optimization of materials, maximizing strength while minimizing volume. This approach has the potential for substantial cost savings, especially in large-scale construction projects.
For added confidence, many high-performance precast concrete connections undergo rigorous prequalification processes. This involves standardized testing protocols to ensure that the systems meet or exceed building code requirements under seismic loading. This gives designers greater assurance in using these innovative connections.
It's important to note that these precast connections are often integrated with other structural systems, like base isolators or damping devices. This comprehensive approach creates a more robust system, further enhancing a building's overall seismic resilience.
Another crucial aspect is the focus on load path continuity in the design of these connections. This ensures that forces are effectively distributed across the entire structural system during seismic activity, helping to maintain stability and preventing the potential for catastrophic collapse.
The development of these connections relies heavily on advanced finite element analysis (FEA). This sophisticated modeling allows engineers to predict the performance of precast connections under various seismic scenarios. FEA provides valuable insights into potential vulnerabilities and helps optimize designs before construction.
While theoretical advancements are promising, real-world performance data further supports the effectiveness of these precast connection systems. Case studies often demonstrate that well-designed precast systems have outperformed traditional systems during actual earthquakes. This data showcases the reliability and effectiveness of these systems in minimizing damage.
Looking ahead, new technologies are constantly emerging in this field. For example, shape memory alloys are being incorporated into precast concrete connections. These alloys have the remarkable ability to automatically return to their original shape after deformation. This self-healing characteristic significantly improves structural performance after an earthquake and accelerates building recovery time.
The ongoing research and development in high-performance precast concrete connection systems hold significant promise. As we strive to build structures that are resilient in the face of seismic events, these innovations have the potential to significantly enhance the safety and longevity of our built environment.
Innovative Beam-to-Wall Connection Techniques for Enhanced Seismic Performance - Hybrid coupled wall systems with replaceable shear links
Hybrid coupled wall systems with replaceable shear links offer a novel approach to enhancing the seismic resilience of structures. This design departs from conventional reinforced-concrete coupled walls by incorporating steel coupling beams that feature replaceable shear links. These links are engineered to yield under seismic loads, absorbing energy and reducing the overall damage to the structure. This concept is particularly appealing due to the ease of replacing the damaged links post-earthquake. This significantly reduces repair costs and minimizes the time required to restore a building to full functionality. The design also boasts innovative beam-to-wall connections that contribute to the system's efficiency and expedite the replacement process after an earthquake. While still in its developmental stages, early studies suggest that hybrid coupled wall systems outperform traditional approaches, making them a promising solution for enhancing structural performance and resilience against seismic events.
Hybrid coupled wall systems with replaceable shear links offer a fascinating approach to improving a building's resilience during earthquakes. These systems consist of two wall piers linked by replaceable steel coupling beams, a configuration that delivers superior seismic performance. The shear links in the system are designed to yield during strong earthquakes, absorbing and dissipating the energy from seismic waves. This ability to yield, rather than fracture, is critical for maintaining structural integrity during strong shaking. The replaceable nature of these shear links offers significant advantages: after an earthquake, only the damaged links need to be replaced, which reduces the cost and complexity of repairs compared to replacing entire wall sections.
The design of the coupling beam itself is crucial for the effectiveness of these hybrid systems. They're often designed with variable stiffness characteristics, meaning their flexibility can be tailored to handle different types of seismic loading. This adaptability provides an added layer of resilience, allowing the structure to adapt to the specific intensity of seismic activity. A notable benefit of this variable stiffness is the ability to distribute forces effectively throughout the structure, minimizing stress concentrations that could lead to localized failures. This multidirectional force resistance is particularly beneficial for large structures, as it helps to ensure a more uniform and robust response to seismic events.
Researchers are continuously testing and refining these systems, seeking to optimize the performance of the shear links and coupling beams. Shaking table tests are being employed to simulate seismic conditions and evaluate the structural behavior of these systems under extreme loading scenarios. These experiments demonstrate the potential benefits of hybrid coupled walls, showcasing their ability to absorb significant energy without catastrophic failure. This data is essential for building confidence in the design and implementation of these systems in real-world projects.
While the concept of hybrid coupled walls is compelling, it's important to remember that these systems are often integrated with other technologies to further enhance structural resilience. For instance, advanced monitoring systems are being integrated to provide real-time data on structural performance during an earthquake. This ongoing monitoring capability allows engineers to track the system's response and adjust strategies as needed to maximize safety and minimize damage. With ongoing research into material science and design optimization, these hybrid coupled wall systems hold significant promise for the future of earthquake-resistant architecture, offering a path towards more resilient and safer structures in seismic zones.
Innovative Beam-to-Wall Connection Techniques for Enhanced Seismic Performance - Rocking walls and metal shear panels for seismic resilience
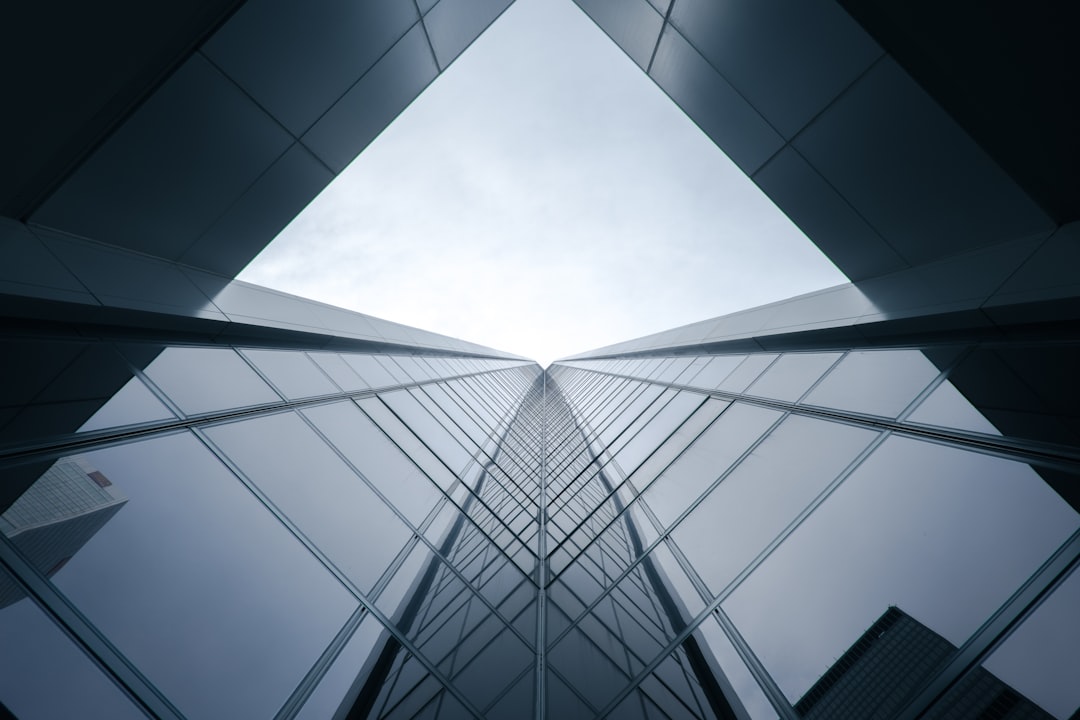
Rocking walls and metal shear panels are emerging as innovative ways to make buildings more resilient to earthquakes. These systems are designed to absorb the energy from seismic events, allowing buildings to essentially "rock" back to their original position after shaking stops. The use of post-tensioned strands in rocking walls is key to their self-centering capability, minimizing lasting damage. The development of self-centering steel plate shear wall systems demonstrates the increasing focus on techniques that control plastic damage and enhance energy dissipation during earthquakes. This trend reflects a significant shift in earthquake engineering, moving beyond simply resisting earthquakes to prioritizing the ability of structures to recover and remain functional after a seismic event. This shift is reflected in the increased use of high-performance materials and hybrid structural systems.
Rocking walls are a relatively new concept in earthquake-resistant design, and they're gaining a lot of attention from researchers and engineers. The idea is that these walls, designed to pivot at their base, act like a giant pendulum during an earthquake. This controlled rocking motion allows them to absorb a lot of the energy from seismic waves, reducing the lateral forces that shake the entire structure.
What makes this even more interesting is the integration of metal shear panels into the rocking wall system. These panels act like shock absorbers, providing energy dissipation through controlled yielding. This is a crucial element in preventing brittle failure, a common concern in traditional wall systems. It's like having a controlled "give" in the system, which helps the building bend without breaking.
The combination of these two elements, rocking walls and shear panels, is showing some promising results. Studies suggest that rocking walls can perform effectively even under severe seismic conditions, and the addition of shear panels enhances this performance. The idea is that rocking walls can reduce the overall seismic response of buildings by up to 50%, which is a substantial improvement over conventional fixed-base structures.
However, we still have a lot to learn. While the initial research is promising, there are many questions about long-term performance, and how these systems would perform in different seismic contexts. We need to conduct more rigorous testing and modeling to validate their effectiveness before widespread adoption.
But the potential for these systems to revolutionize earthquake-resistant design is undeniable. They offer the promise of safer, more resilient buildings that can withstand even the most powerful earthquakes. Imagine a future where buildings can bounce back from a disaster with minimal damage and disruption, allowing for faster recovery and reduced rebuilding costs. It's a vision worth pursuing, and with further research, we might be closer than we think.
Innovative Beam-to-Wall Connection Techniques for Enhanced Seismic Performance - Spliced walls using advanced connection techniques
Spliced walls represent a major step forward in seismic design, offering enhanced resilience against earthquakes. Studies show that these walls resist bending-shear failures effectively, with no significant difference in performance between prefabricated and cast-in-situ connections. Using self-compacting concrete in double spliced shear walls has proven particularly effective, significantly boosting seismic performance compared to traditional methods. This promising progress highlights the potential of further research and development of spliced walls, offering a path towards safer and more resilient structures in earthquake-prone areas.
Spliced walls, where sections of wall are joined together, are becoming more sophisticated in their design to improve seismic performance. This advancement is fueled by a focus on how these connections can be used to better manage the energy of earthquakes. Researchers are looking at various methods for splicing, including the use of shear keys or post-tensioning techniques, as ways to enhance energy dissipation during an earthquake. This dissipation can help to prevent damage by minimizing stress build-up and reducing the likelihood of catastrophic failure.
There's also a trend toward incorporating flexibility into these spliced wall systems. This flexibility is achieved by designing connections that allow for controlled movement. This controlled movement allows the structure to more effectively absorb seismic forces and then return to its original position after the earthquake. This concept of "self-centering" is crucial for minimizing damage and ensuring that the building is ready for use after a seismic event. The way in which the connections are designed also influences how the loads are spread throughout the wall, aiming for more uniform distribution. This minimizes the concentration of stress at any single point, reducing the risk of localized failures that could weaken the entire structure.
One of the benefits of these spliced walls is that they are often designed for easy replacement of individual components. If a part of the wall is damaged during an earthquake, it can be swiftly replaced, limiting the downtime and minimizing the cost of repairs. Spliced walls also have the advantage of being easily integrated with other technologies that improve seismic performance, such as base isolators. This integration creates a synergistic effect that further strengthens the entire building and provides added safety against earthquakes.
Additionally, the modularity of these systems makes for faster installation. Spliced wall components can be pre-assembled off-site, which speeds up construction. This approach can help to reduce overall project timelines, especially in areas where time is a factor in the building process.
By using optimized designs, engineers are able to create spliced walls that are lighter and require less material than conventional structures. This has the added benefit of reducing construction costs, especially for larger projects. Spliced walls can also be designed to withstand various seismic wave configurations, making them effective in diverse environments.
While the concept of spliced walls has great potential, their application is being further refined through computational modeling and shake table testing. These methods help engineers understand how the walls will perform under different seismic conditions. Spliced walls are also increasingly meeting, and even exceeding, building codes, which are continuously becoming more stringent in their requirements for seismic resilience.
Revolutionize structural engineering with AI-powered analysis and design. Transform blueprints into intelligent solutions in minutes. (Get started for free)
More Posts from aistructuralreview.com: