Revolutionize structural engineering with AI-powered analysis and design. Transform blueprints into intelligent solutions in minutes. (Get started for free)
Optimizing Water-Curing Techniques for Enhanced Concrete Durability A 2024 Update
Optimizing Water-Curing Techniques for Enhanced Concrete Durability A 2024 Update - Advanced Automated Curing Systems for Uniform Hydration
Advanced automated curing systems offer a promising approach to achieving more uniform and controlled concrete hydration. Unlike traditional manual methods, which often struggle to maintain consistent moisture and temperature, these systems can precisely regulate these crucial factors. This precision minimizes the risk of early-age cracking, a common issue in manually cured concrete that significantly compromises durability.
These systems are engineered with a focus on real-time monitoring of the hydration process. This allows for a consistent curing environment across different concrete mixtures and sizes, ensuring that each batch achieves optimal hydration. The ability to carefully manage hydration through automation can positively impact the concrete's microstructure, contributing to enhanced strength and a longer lifespan.
The move towards automation in concrete curing signifies a shift towards greater efficiency and reliability. By incorporating these technologies, the construction industry can achieve a higher level of consistency and quality in the concrete it produces, ultimately leading to more durable and resilient structures. While still a developing field, the potential benefits of automated curing are undeniable and warrant further investigation and implementation.
Modern automated curing systems are increasingly reliant on IoT sensors to meticulously monitor temperature and moisture in real-time. This constant monitoring allows for fine-tuned adjustments, optimizing the hydration process and potentially pushing concrete strength to higher levels. Research has demonstrated that even slight inconsistencies in moisture during curing can have a substantial effect on concrete's tensile strength, with differences exceeding 20% in uncontrolled scenarios. Some of the more sophisticated systems automate water vapor adjustments, which is especially beneficial for smaller projects ensuring uniform curing without wasteful water consumption. We're starting to see the emergence of computer algorithms that predict ideal curing conditions based on external environmental factors, leading to potential reductions in energy use while simultaneously maximizing performance. Integrating technologies like infrared curing lamps into automated systems offers an interesting approach. They can rapidly raise the concrete's surface temperature, accelerating the hydration process without compromising the material's integrity. It's fascinating to see that many of these automated systems incorporate feedback loops that constantly evaluate the curing progress. This allows for immediate modifications, leading to a significant improvement in the final concrete's durability. Intriguingly, studies have indicated that concrete cured using these automated systems demonstrate enhanced resistance to freeze-thaw cycles, which suggests an increase in its overall lifespan when compared to traditional methods. The precision afforded by these automated systems potentially minimizes shrinkage cracks, a frequent issue with conventional curing that can undermine the structural stability of concrete. Some advanced technologies are employing dielectric measurement techniques to gain a more granular understanding of moisture levels throughout the curing process, enabling a more accurate assessment of hydration. And while complex in function, many automated systems incorporate fail-safe mechanisms. This ensures that if a monitored parameter drifts beyond predefined limits, the system automatically triggers corrective actions to protect concrete quality.
Optimizing Water-Curing Techniques for Enhanced Concrete Durability A 2024 Update - Self-Curing Concrete Reduces External Water Dependency
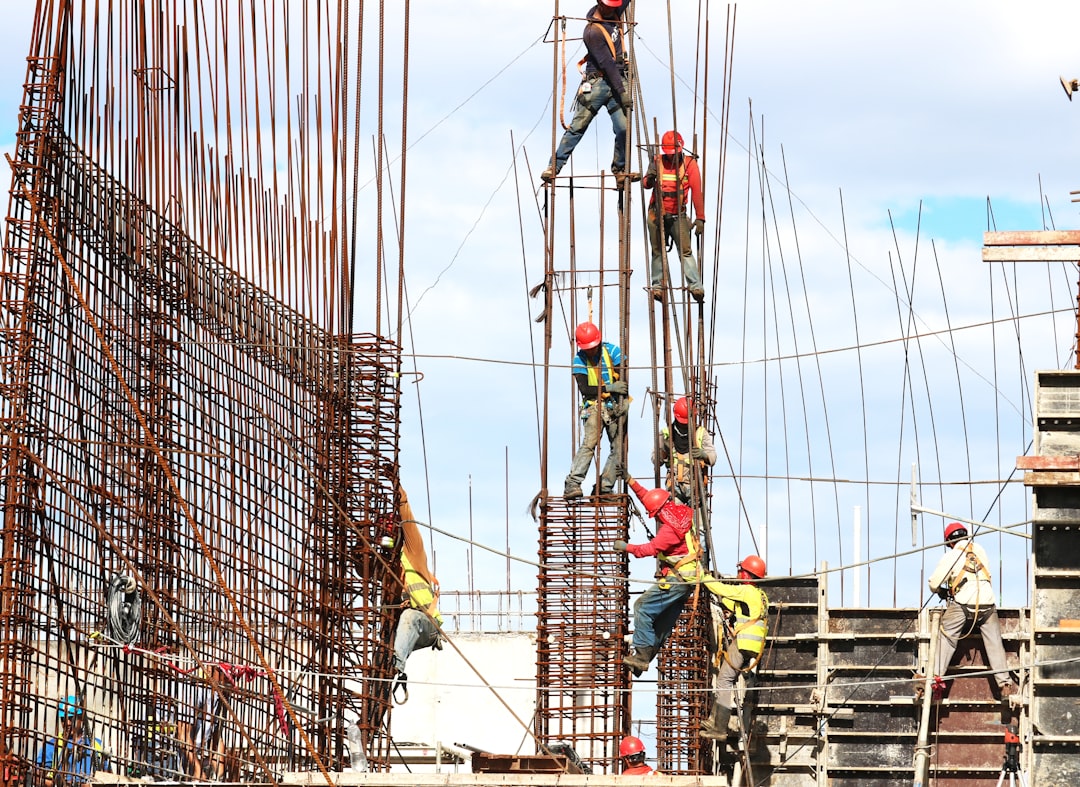
Self-curing concrete represents a notable shift in concrete technology, aiming to minimize the reliance on external water for proper curing. It achieves this by harnessing internally retained moisture to facilitate the hydration process, effectively curing from within. This internal hydration helps mitigate the issues of autogenous shrinkage and self-desiccation, both of which can negatively impact concrete durability.
The effectiveness of self-curing concrete is often enhanced by incorporating specific chemical agents, such as polyethylene glycol (PEG), to retain moisture during the curing period. Additionally, the use of supplementary cementitious materials (SCMs) can contribute to improved early strength and reduced permeability, ultimately contributing to enhanced resistance to the effects of environmental factors.
A key advantage of self-curing concrete lies in its applicability to situations where external water availability is limited or problematic. This makes it a promising solution for construction projects in challenging environments or regions experiencing water scarcity. Given the growing global construction industry and its environmental impact, self-curing concrete aligns with broader industry trends aimed at developing more sustainable construction practices. While promising, it's crucial to acknowledge that the technology is still evolving and research continues to explore the optimal application of these internal curing mechanisms.
Self-curing concrete operates by incorporating materials that internally store and release water, effectively reducing the need for external water sources during the curing phase. This internal hydration process seems to help manage the challenges of environmental variations that can impact traditional curing.
It's intriguing that research suggests self-curing concrete can achieve comparable, if not improved, hydration compared to standard methods. This is particularly interesting given the potential for reducing water loss through evaporation, as it appears to maintain optimal moisture levels without continuous external application or monitoring.
The concept is promising, with reports indicating that it can boost compressive strength, possibly by as much as 30% compared to conventional techniques. This implies a potential for increased load-bearing capacity in structures. Early-stage cracking, often linked to moisture loss, is a common concern, and self-curing agents show potential to minimize this risk by retaining necessary moisture.
One of the practical benefits seems to be a potential reduction in labor associated with traditional water curing. This streamlining of construction processes could free up labor for other crucial tasks without sacrificing concrete quality. It's surprising that the technology can maintain sufficient moisture levels even in dry environments, which traditionally pose a significant challenge due to rapid moisture loss.
Some formulations are designed to provide inherent impermeability, further mitigating the risk of water intrusion which is a major factor in long-term concrete degradation. The potential for construction in water-scarce regions or areas with limited access to water is quite valuable, particularly for projects in regions with resource limitations.
It's worth noting that the effectiveness of self-curing can be potentially improved by the addition of superabsorbent polymers. These can essentially act as internal reservoirs, soaking up a considerable amount of water for later release, potentially enhancing the consistency of the hydration process. Interestingly, evidence indicates that this method not only optimizes hydration but can also enhance long-term performance in demanding environments, suggesting a practical application in infrastructure projects in challenging climates. While promising, ongoing research and field trials are crucial to assess its true potential and limitations in diverse applications.
Optimizing Water-Curing Techniques for Enhanced Concrete Durability A 2024 Update - Internal Curing Techniques Using Water Reservoirs
Internal curing using water reservoirs has emerged as a crucial technique, especially for high-performance concretes like ultra-high performance concrete (UHPC). These methods introduce materials that act as internal water sources, thereby improving the hydration process within the concrete. This is particularly important because it helps manage issues like the rapid shrinkage that occurs early in the concrete's life, something that traditional surface-based curing methods often fail to address effectively. The ability to provide a readily available internal source of moisture is beneficial in reducing the negative impact of self-desiccation, a phenomenon that can severely compromise a concrete's long-term performance, especially in mixes designed with lower water-to-binder ratios. Furthermore, recent advancements include the use of specific chemicals, such as carbamide solutions, and polymers that can store water, boosting the efficiency of internal curing, ultimately leading to better hydration and improved mechanical strength within the concrete. As the demands on concrete infrastructure continue to grow, incorporating these internal curing approaches is becoming increasingly important for building stronger, more sustainable structures in a variety of conditions. While promising, there is still some debate about the optimal use and limitations of these approaches.
Internal curing, a technique involving incorporating a water reservoir within the concrete mix, offers a promising approach to enhance hydration, particularly in concrete formulations with lower water-to-binder ratios. This strategy appears particularly beneficial for ultra-high-performance concrete (UHPC), where it can effectively counteract the significant early-age autogenous shrinkage that often accompanies these high-strength mixes. Conventional external curing methods, which primarily focus on surface moisture, might not be sufficient for such high-performance concretes due to their limited penetration depth. The water reservoirs provided by internal curing can potentially reduce the negative effects of self-desiccation, a process where the concrete dries out from within, especially important in UHPC where shrinkage can be substantial within the first 24 hours. Some concrete mixtures may require a considerable portion of internal curing water—up to 50% in some cases—to effectively address this issue.
Several materials can serve as these internal reservoirs, such as perforated cenospheres, which, while providing the water source, might also help reduce chemical shrinkage in the cement paste. Studies suggest that internal curing, especially when conducted with a cool water reservoir, effectively delivers water from within the concrete, which could potentially lead to a reduction in early-age cracking and overall improvements in the hydration process. While the cost of incorporating internal curing agents appears relatively low (around $0.11 per unit), it's still a factor in cost-benefit analyses for various projects. Recent research focuses on using carbamide solutions as internal curing agents, which might enhance the cement paste's stiffness by forming calcium carbonate. The incorporation of these internal curing mechanisms seems critical to maximizing the durability and mechanical properties of concrete structures, particularly in challenging environments where moisture management is critical. This approach could be particularly important in structures subject to harsh weathering conditions or demanding loading scenarios.
Optimizing Water-Curing Techniques for Enhanced Concrete Durability A 2024 Update - Ultrahigh Performance Concrete with Fiber Reinforcement
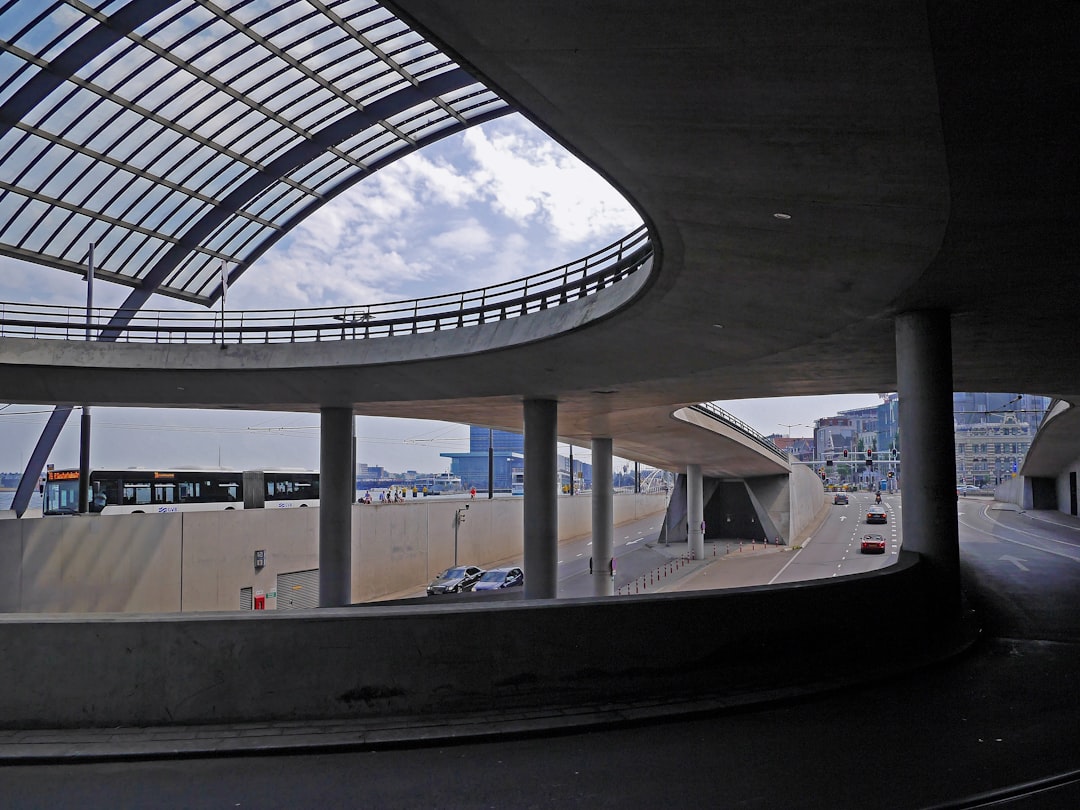
Ultra-high-performance concrete with fiber reinforcement (UHPFRC) marks a significant leap in concrete technology, offering exceptional strength and durability compared to traditional concrete. It achieves this by combining a high-strength concrete matrix with the reinforcement provided by embedded fibers. This innovative combination results in a material that excels in withstanding challenging environmental conditions like extreme temperature fluctuations and corrosive substances.
However, achieving the remarkable compressive strengths—often exceeding 150 MPa—characteristic of UHPFRC necessitates specialized curing methods. These typically include techniques like applying heat and extensive vibration, which are not standard for traditional concrete. Optimizing the mix design of UHPFRC is crucial to realizing its full potential. This involves carefully controlling the water content, often keeping it below 0.2, and maximizing the use of high-performance components like silica fume, specialized fibers, and superplasticizers.
The superior mechanical characteristics of UHPFRC make it ideal for structural applications demanding high load-bearing capacities. It's also particularly attractive for projects in challenging environments due to its resistance to degradation. The field of UHPFRC is continuously evolving, with ongoing research actively exploring different types of fibers and even nanomaterials to further refine the material's properties and expand its utility in modern engineering. These innovations have the potential to unlock even greater possibilities for UHPFRC in the future of construction. While its use is still developing, the prospects for UHPFRC seem to be significant.
Ultra-high-performance fiber-reinforced concrete (UHPFRC) represents a significant advancement in cementitious materials, combining a high-performance concrete base with embedded fibers. Its development, starting in the mid-1990s, has yielded a material with notably superior mechanical properties and improved durability compared to traditional concrete. Achieving the impressive compressive strengths—often exceeding 150 MPa—requires specialized curing, typically including heat curing and vigorous vibration.
Optimizing UHPFRC involves a careful balancing act. It's crucial to minimize water content, typically to below 0.2, while utilizing a high volume of cement, silica fume, fibers, and superplasticizers. This approach is critical to its high performance. The enhanced mechanical characteristics of UHPFRC stem from a refined microstructure, reduced porosity, and a homogeneous mix, leading to superior durability and overall performance. The formulation draws inspiration from the dense packing theory, focusing on maximizing the density of the composite and optimizing its structural integrity.
Recent research emphasizes refining UHPFRC mix designs to further unlock its potential across a wider range of engineering applications. One of its key advantages is the ability to significantly reduce the self-weight of structures while improving their span capabilities, making it a compelling choice for modern construction. UHPFRC also provides improved resistance to freeze-thaw cycles and acidic attacks, making it well-suited to demanding environmental conditions. The potential for UHPFRC is further expanded by ongoing exploration of various fiber and nanomaterial types that can potentially refine its properties and broaden its applications.
However, UHPFRC isn't without its challenges. While offering long-term cost savings due to enhanced durability and reduced maintenance requirements, the initial material cost of UHPFRC is often higher than standard concrete. Also, the specialized curing methods necessary to achieve optimal performance can require a higher level of control and management than traditional concrete curing. Despite these complexities, the research community continues to invest in UHPFRC research, working towards understanding the limits and opportunities of this advanced material for a wide variety of construction applications. The goal is to refine the design process, making UHPFRC more versatile and accessible to engineers.
Optimizing Water-Curing Techniques for Enhanced Concrete Durability A 2024 Update - Bacterial Self-Healing Methods for Crack Repair
Concrete's susceptibility to cracking can significantly reduce its lifespan and structural integrity. A relatively new approach to mitigating this issue is the use of bacteria for self-healing. These bacterial-based methods rely on a process called Microbial Induced Carbonate Precipitate (MICP) where bacteria, when activated by water entering a crack, produce minerals that effectively fill and seal the damage.
Experiments have confirmed the viability of this approach, showing that certain bacteria, such as Sporosarcina koreensis and Bacillus subtilis, can enhance the healing process and overall concrete durability. In trials, bacterial concrete healed cracks as wide as 0.46 mm, while traditional concrete methods managed only 0.18 mm under similar conditions. This performance is even more impressive when considering the reduced strength loss observed in bacterial concrete, only 10.7% compared to 16.4% in conventional concrete after 28 days of curing.
To further improve healing capabilities, techniques like encapsulating the bacteria in hydrogels have been developed. These encapsulated spores remain dormant until triggered by a crack, enabling them to address larger and potentially more damaging cracks. Although these self-healing techniques are showing promising results, more research is needed. The optimal balance of effectiveness, cost, and applicability across different concrete formulations and environmental factors remains unclear. For instance, while a longer curing period can be detrimental to the durability of both conventional and bacterial concrete, it is worth noting that bacterial concrete shows better performance overall when extended curing periods are employed.
Concrete incorporating bacteria offers a compelling approach to enhancing its durability by leveraging a natural crack-repair mechanism. Certain bacteria produce calcium carbonate, a substance that can effectively seal cracks when activated by water intrusion. This approach could potentially lead to significant reductions in the frequency and cost of traditional repairs.
The beauty of this approach lies in the fact that the bacteria, in the form of dormant spores, can persist within the concrete for long periods until triggered by the presence of water, typically caused by a crack forming. This means that the self-healing mechanism is available throughout the life of the structure. Preliminary studies have revealed that these bacterial-based systems can repair cracks up to 0.46 mm wide in a matter of days, substantially faster and likely more economically than conventional repair methods.
In various trials, bacteria like *Sporosarcina koreensis* and *Bacillus subtilis* have shown promising results in improving the overall performance of concrete, especially in high-strength varieties. While bacterial concrete initially exhibits a slight reduction in strength, it consistently demonstrates a slower rate of degradation over time compared to conventional concrete. Interestingly, researchers have discovered that encapsulating the bacteria in hydrogels can lead to a more effective healing of larger cracks.
Further, incorporating techniques like surface coatings can complement bacterial-based self-healing strategies by providing an initial layer of protection, potentially reducing the frequency of bacterial activation. However, it's interesting that studies indicate both bacterial and conventional concretes experience a gradual decline in durability over extended curing periods. Yet, bacterial concrete appears to age gracefully, showing a more gradual deterioration.
While these self-healing agents show promise for addressing existing cracks, it is important to recognize that they have limitations. While they can address many cracking issues, they may not be as effective for very large or complex cracks that would typically require more structural intervention. Furthermore, their effectiveness is tied to the conditions in which they exist, so the environment needs to be considered in the design process for optimizing performance.
It appears that the inclusion of additional nutrients, like peptone and yeast extract, might improve the effectiveness of bacterial healing in harsher conditions such as in environments containing corrosive substances. These added nutrients could help the bacteria thrive in challenging environments and continue to provide crack-repairing abilities. The long-term implications of such self-healing approaches are fascinating. They hold the potential to improve the structural resilience of our infrastructure by addressing the challenges of cracks and corrosion that typically occur in concrete structures.
However, the transition of bacterial self-healing methods to large-scale applications isn't without its challenges. Maintaining uniform mixing of the bacteria throughout the concrete and managing the microbial activity during the curing process are significant factors that need further refinement. Moreover, the ideal strain of bacteria, optimum environment conditions, and appropriate concentration within the concrete are areas of ongoing research to broaden the application possibilities. Nonetheless, the potential benefits are substantial, and research in this emerging field continues to push the boundaries of what's achievable in concrete durability.
Revolutionize structural engineering with AI-powered analysis and design. Transform blueprints into intelligent solutions in minutes. (Get started for free)
More Posts from aistructuralreview.com: