New Insights Comparative Analysis of Shear Strength in High-Performance Steel Grades for Structural Applications
New Insights Comparative Analysis of Shear Strength in High-Performance Steel Grades for Structural Applications - Comparative Analysis of Yield Strength Across HPS Grades
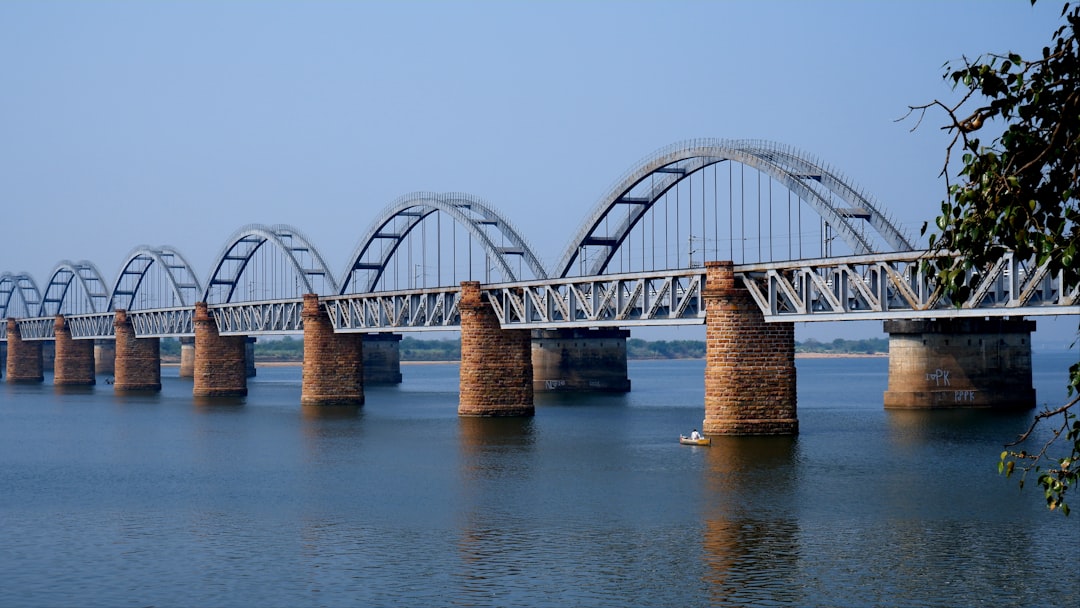
Examining yield strength across different HPS grades reveals a notable shift in structural steel capabilities. Traditional steel grades were limited to yield strengths around 36 ksi and 50 ksi, whereas the development of HPS has introduced grades with minimum yield strengths significantly higher, exemplified by HPS 485W at 485 MPa (approximately 70 ksi). This leap in yield strength provides a foundation for enhancing structural performance, particularly in applications demanding higher load capacity and durability, like bridges. Moreover, advancements in HPS development have addressed past concerns regarding high-strength steels, showing enhanced ductility and energy absorption under stress, mitigating the risk of brittle failures that plagued earlier high-strength options. The nuanced differences in yield strength between HPS grades are critical for engineers to comprehend, informing optimal material selection and structural design in diverse applications.
Examining the yield strength values across various HPS grades reveals a wide spectrum of performance characteristics. Some HPS grades now surpass 700 MPa in yield strength, a substantial improvement compared to conventional grades like S235. Achieving these high strength levels often involves specific alloying techniques, incorporating elements like niobium and vanadium to modify the microstructure. Maintaining desirable ductility alongside this increased strength is crucial for structural integrity.
Interestingly, some HPS grades show superior resistance to yield strength reduction under elevated temperatures, hinting at potential for use in scenarios requiring fire resistance. Microstructural analysis reveals a trend toward finer grain structures like bainite, compared to the traditional ferrite-pearlite found in older steels. This microstructural evolution is intrinsically linked to the observed enhancement in yield strength.
The manufacturing processes themselves can influence the resulting yield strength. Processes such as quenching and tempering can lead to exceptional strength levels, potentially exceeding 1000 MPa in certain cases. However, it is becoming clear that the yield strength variation across different HPS grades is tied to the grain size. The Hall-Petch relationship, which connects grain size to strength, highlights this.
We also observe a connection between yield strength and fatigue performance. It seems that higher yield strength often translates to improved fatigue resistance, a valuable attribute for structural elements subjected to cyclic loading. But it's crucial to acknowledge that the pursuit of high yield strength should not overshadow the importance of toughness. Some high-yield HPS grades demonstrate a tendency towards brittleness at lower temperatures, highlighting the need to select HPS grades with specific consideration for the operating environment.
Furthermore, optimizing the heat treatment process is essential in achieving the desired yield strength properties in newly developed HPS grades. Fine-tuning temperature and cooling rates are vital to tailoring the material's mechanical properties. The relationship between yield strength and the overall performance in structural applications is multifaceted. While yield strength is a critical indicator, ductility and toughness are equally important when evaluating the suitability of an HPS grade. A comprehensive approach is needed to ensure a material’s ability to handle unforeseen stresses or impacts. The continuing development of HPS is a testament to the ongoing pursuit of higher strength in structural materials, and understanding this spectrum of properties remains a central theme within the field.
New Insights Comparative Analysis of Shear Strength in High-Performance Steel Grades for Structural Applications - Impact of Shear Reinforcement on Structural Element Performance
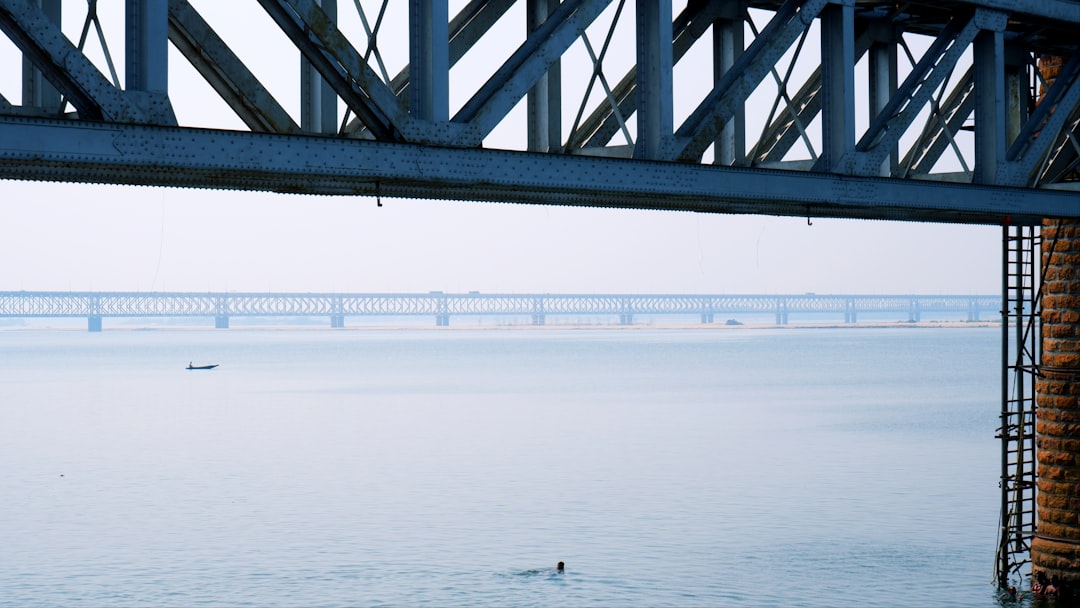
The role of shear reinforcement in influencing the performance of structural elements is a key factor in ensuring their stability and strength. Modifications to the design of shear reinforcement, such as adjusting its geometry, angle, and spacing, have shown promise in improving both shear and flexural behavior. Furthermore, incorporating high-performance materials and optimizing the ratios of reinforcement can significantly improve the structural integrity of reinforced concrete elements, especially when subjected to dynamic loads.
However, predictive models for various reinforced structural members like columns and joints have shown significant discrepancies in their ability to accurately assess performance, sometimes underestimating or overestimating actual behavior. This underscores the importance of carefully considering reinforcement placement and the potential tradeoffs that may arise. Achieving optimal shear strength while avoiding the potential for brittle failure continues to be a major challenge in the design of high-performance structures. The design methodologies for advanced materials and structural forms will likely need to address these issues as engineering practices continue to evolve.
Researchers have developed models to estimate the shear capacity of reinforced concrete (RC) beams, taking into account the amount and arrangement of shear reinforcement. These models are particularly important for wider or shallower beams. Interestingly, when we examine deeper RC beams, higher concrete strength, increased reinforcement ratios, and using steels with higher yield strengths all lead to improved shear capacity. However, the ratio of the beam's depth to its width has a negative impact on shear strength, something to consider when designing such elements.
Predicting how various reinforced structural members, such as columns and beam-column joints, will perform under shear loading has proven to be challenging. Different prediction methods have varying degrees of accuracy, with some overestimating or underestimating the actual behavior. This highlights a need for more robust and reliable methods to capture the complexities of shear behavior in these more intricate structures.
In China, design guidelines suggest a limit on the average nominal tensile stress of shear wall sections. It's advised that this stress shouldn't exceed twice the tensile strength of the concrete core. However, they also allow for increasing this stress if adequate embedded steel is incorporated. This rule underscores how shear reinforcement interacts with the inherent strength of the concrete, but it's unclear how generally applicable this specific guideline is to a global design context.
The ways in which we strengthen standard reinforced concrete beams have been extensively studied. Using high-performance concrete (HPC) or adding extra reinforcement are effective methods for improving beam performance. However, we've found that a risk of brittle failure under shear loading exists. This is not a new observation but remains a consideration when designers leverage these strengthening techniques.
Researchers have created comprehensive empirical equations to predict the shear strength of reinforced concrete deep beams with greater accuracy. One particular effort analyzed data from 198 deep beams from multiple studies to achieve this. While these new formulations are a helpful step towards more precise predictions, there's a constant need for continuous research to validate these methods for a wider range of conditions and beam configurations.
The behavior of ultrahigh-performance steel fiber-reinforced concrete beams under shear has also been a focus of study. The researchers concentrated on load-bearing capacity and shear strength, particularly in cases where the shear span-to-depth ratio is low. These types of beams represent an interesting material frontier, and it's not entirely clear how the inclusion of steel fibers truly alters the shear-strength characteristics of these beams. More research is likely needed to build out a wider body of understanding here.
It's become apparent that modifying the traditional steel reinforcement within RC beams—changing their shape, inclination, or spacing—can significantly enhance shear and flexural performance. Engineers are continually finding clever ways to improve the efficacy of shear reinforcement design, and these kinds of innovations push the limits of what's possible in concrete design.
Studies show that different arrangements of shear reinforcement have a profound effect on the shear and flexural behavior of concrete beams. This makes sense intuitively, but it's good to have it supported by evidence. This realization helps to explain the need to carefully consider how the reinforcement is deployed during the design phase.
The development of new design methods for shear in concrete beams reinforced with ultra-high-performance steel bars holds promise for enhancing the behavior of structural elements under dynamic loads. This advancement speaks to the increasing awareness of how HPS grades can be more effectively implemented in applications involving more complex loading scenarios.
It seems there's a general need for further investigation of how shear reinforcement should be handled in design codes. These findings reveal gaps in industry standards that could affect design approaches and compromise safety evaluations. This isn't to say that current standards are necessarily 'wrong', but rather that the rapid pace of change in materials and design practice has created a need to update the regulatory framework.
New Insights Comparative Analysis of Shear Strength in High-Performance Steel Grades for Structural Applications - Advancements in Predictive Modeling for Shear Capacity Analysis
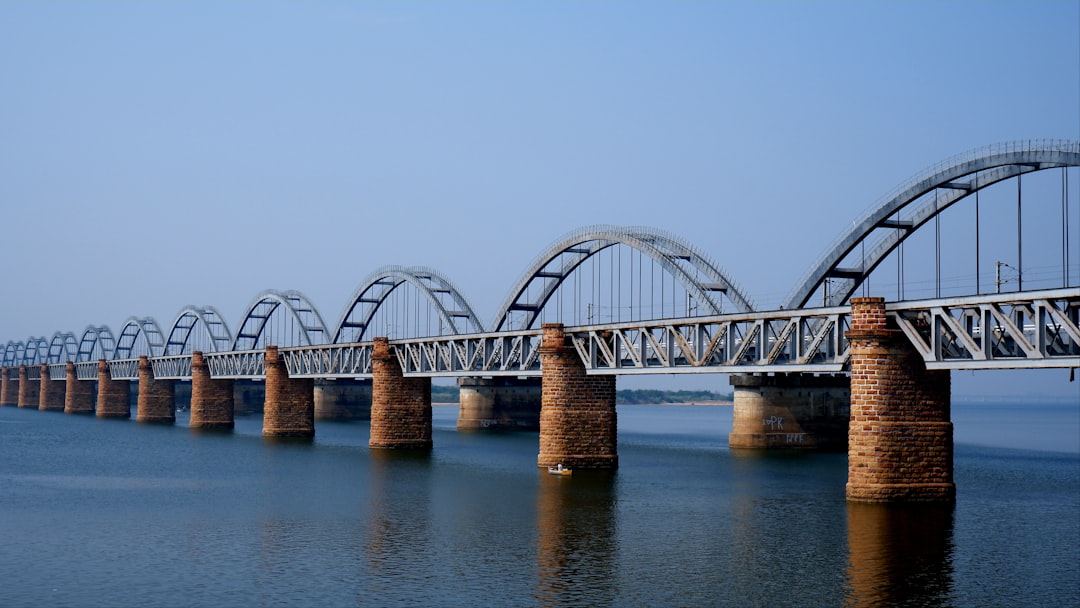
The field of shear capacity analysis has seen significant strides with the development of new predictive modeling techniques. These advancements are driven by a need for more precise and reliable methods to understand the complex behavior of structures under shear loading. Researchers have explored using artificial intelligence, particularly artificial neural networks (ANNs), to predict shear behavior in structures like concrete-encased steel (CES) beams. This approach stems from experimental work involving high-strength steel, offering a potential path toward improved understanding and prediction.
In addition, researchers have refined empirical methods to better predict the shear strength of reinforced concrete deep beams. These refinements include incorporating variables like the ratio of shear span to beam depth and the arrangement of reinforcement. This has led to more accurate empirical equations, helping to enhance existing structural design codes.
However, the complexity of shear behavior in structures, particularly those utilizing modern high-performance materials, remains a challenge. Researchers are consistently encountering scenarios that demonstrate the limitations of current predictive models. As a result, there's a clear need to revise and update design codes to ensure they adequately reflect the latest understandings of shear behavior. Continued development and refinement of predictive modeling approaches will be crucial for adapting to the use of advanced materials and structural designs, guaranteeing structural safety and reliability.
Recent progress in predicting shear capacity has seen the rise of machine learning techniques. These algorithms are proving useful in understanding complex structural behaviors that traditional methods often struggle to capture, leading to much more accurate predictions. In some cases, predictive models can now incorporate live data from structures. This allows for adaptive estimations of shear capacity based on real-world loading, departing from the purely theoretical analyses that were once the standard.
Further advancements have come from investigating the nonlinear aspects of high-performance steel properties. This allows predictive models to consider how shear behavior can vary due to material imperfections or variations in manufacturing. The incorporation of powerful computational methods like finite element analysis (FEA) has greatly improved our understanding of localized shear failures. This gives engineers detailed insights into how HPS materials respond in diverse loading situations.
It's been surprising to see that the shear capacity's relationship with loading rates suggests higher loading rates can significantly improve the shear strength of HPS beams. This finding requires deeper exploration within predictive models. New empirical connections have been developed linking shear capacity to the microstructure of HPS. These include grain size and are leading to more sophisticated predictive modeling informed by material science.
Some cutting-edge models suggest that specific reinforcement configurations can improve shear capacity. This challenges previous assumptions that simply increasing the quantity of reinforcement is sufficient. Recent work highlights how predictive models are successfully integrating environmental conditions like temperature and humidity. These factors can influence structural element shear behavior in real applications.
A rising trend is the use of probabilistic methods in predictive modeling. These are aimed at capturing the uncertainty in material properties and loading conditions. This represents a shift away from the deterministic models traditionally used in structural engineering. Interestingly, regulatory changes within engineering standards are beginning to acknowledge these advanced predictive models. This hints that incorporating advanced analysis methods could soon reshape how shear capacity is considered in future structural design guidelines. It will be fascinating to see how this interplay of theoretical and regulatory advancements will affect practice.
New Insights Comparative Analysis of Shear Strength in High-Performance Steel Grades for Structural Applications - Shear Resistance Comparison Between High-Strength and Low-Strength Steel
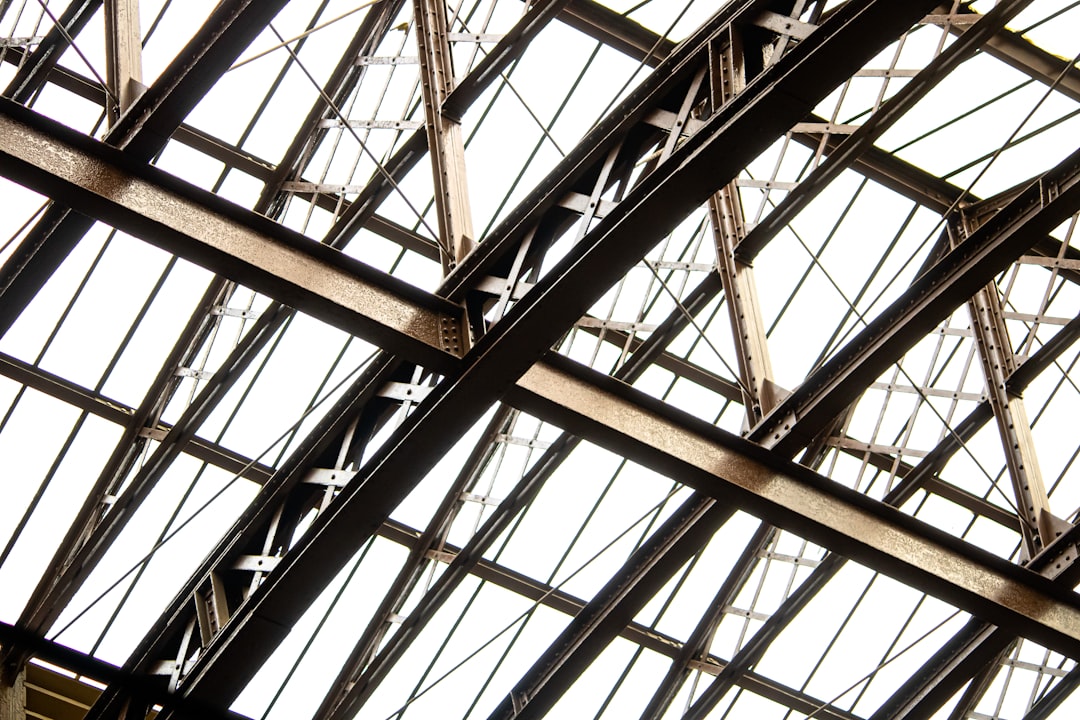
When comparing the shear resistance of high-strength steel (HSS) and low-strength steel (LSS), significant differences emerge that have implications for structural design. HSS, with its yield strength exceeding 460 MPa, offers improved mechanical characteristics compared to LSS. This translates to higher shear capacities and superior performance under various stress conditions. A key aspect of shear strength in steel structures is the tendency of the web to yield before the flanges, making it a critical factor in determining the overall shear capacity. The intricate nature of shear mechanics becomes particularly relevant when dealing with high-performance steels. Given the continuous progress in materials science and design techniques, the choice between HSS and LSS increasingly influences how engineers approach ensuring structural soundness and optimizing overall performance. The understanding of these variations in shear behavior is crucial for efficiently managing load transfer and promoting safety within steel structures.
High-strength steel's shear resistance is intricately tied to its internal structure, with processes that refine the grain size appearing to enhance shear strength. This sensitivity to manufacturing nuances can lead to substantial performance variations, even with seemingly small changes.
Low-strength steel often exhibits greater ductility at the point of failure when compared to high-strength alternatives. This can result in brittle failures during shear loading in some situations, underscoring the critical role of selecting materials that match the specific application.
Intriguingly, shear strength doesn't always increase in direct proportion with yield strength; there can be a nonlinear relationship. This emphasizes the need for engineers to conduct detailed modeling and testing in real-world situations.
Some studies have found that strategic arrangements of shear reinforcement can produce a greater shear strength compared to simply increasing the overall quantity of reinforcement. This challenges long-held design assumptions.
The impact of loading rates on shear strength is noteworthy. It appears that higher loading rates can improve the shear capacity of high-strength steels because they don't have time to yield before failure, suggesting a shift towards dynamic considerations in design.
Recent applications of machine learning are allowing predictive models to incorporate real-time data, which can enhance the accuracy of shear strength predictions for structures under varying loads throughout their lifespan.
Researchers have discovered that the way stress is distributed within reinforced concrete beams can significantly impact shear strength. This suggests that a uniform distribution of reinforcement isn't always optimal and requires meticulous design choices.
High-strength steels may exhibit more pronounced susceptibility to temperature changes when compared to their lower-strength counterparts, influencing their shear behavior under extreme conditions. This underlines the importance of rigorous thermal testing for structural materials.
The requirements for effective shear reinforcement have evolved. For example, it appears that employing a staggered arrangement of stirrups can transfer shear forces more effectively than traditional designs, opening the door for further innovative design solutions.
Many assessments of shear performance rely on existing codes that were developed without consideration for advanced high-performance materials. This highlights the increasing urgency for updated regulations that accurately represent the advancements in steel technology.
New Insights Comparative Analysis of Shear Strength in High-Performance Steel Grades for Structural Applications - Integration of Steel Fibers in Ultra-High-Performance Concrete for Shear Strength
The use of steel fibers within ultra-high-performance concrete (UHPC) presents a promising way to increase shear strength in structures. Research has shown that including steel fibers noticeably enhances the shear capacity of beams, especially those with a short shear span compared to their depth. This improvement is due, in part, to a change in the way the beams fail and to the increase in the maximum shear load they can withstand. Beyond simply improving the mechanical characteristics of the UHPC, steel fiber reinforcement also benefits concrete-filled steel tubes. This is because the fibers help prevent premature buckling and keep the concrete under a favorable compression load on all three sides. Beyond basic fiber inclusion, the shape of the fibers and even the addition of tiny nanofibers can further influence the material’s performance. These findings open up opportunities for using UHPC in different structural situations like precast composite beams (concrete and steel) and shear walls. While these uses are interesting, it's still unclear how much UHPC can be utilized in a variety of designs. More investigation in this area would be helpful.
The integration of steel fibers within ultra-high-performance concrete (UHPC) has proven to be a promising approach for enhancing its shear strength, particularly in structural elements like beams that experience loads with relatively low shear span-to-depth ratios. Experimental work on UHPC beams reinforced with steel fibers has provided valuable insights into their ultimate shear behavior, including how the fiber content affects shear strength and failure mechanisms. Notably, it appears that these fibers can effectively bridge cracks within the concrete, which improves the material's ability to manage tensile stresses and delays the onset of failure.
There's a fascinating relationship between the fiber's shape and the material's shear response. The way steel fibers are shaped, whether straight, hooked, or crimped, impacts the shear performance. Hooked fibers, for example, tend to provide better anchorage within the concrete matrix due to their unique design, and thus enhance the material's capacity to absorb energy during shear loading. This mechanical interaction between the fiber and the UHPC matrix is further boosted by surface treatments applied to the fibers, which improves the interfacial adhesion. This enhanced bonding contributes to both increased ductility and greater shear resistance of the resulting UHPC composite.
Interestingly, it's not just about adding more steel fibers; the volume fraction of fibers appears to play a role. Research indicates that once the fiber volume fraction surpasses a certain threshold, often around 1%, there are diminishing returns in terms of shear strength improvement. This signifies that there's an optimal point for the fiber content in UHPC design to avoid unnecessary material and weight increases.
The role of steel fibers extends beyond merely increasing the shear capacity. They also influence how UHPC fails. While standard concrete tends to fail in a brittle manner with limited warning, the presence of steel fibers in UHPC seems to promote a more ductile failure. This offers potential advantages in terms of providing early indications of structural distress, potentially giving time for intervention before complete collapse.
Beyond their impact on shear strength, steel fibers also contribute to improved post-cracking behavior in UHPC, which enhances the overall resilience of the material. This is especially beneficial in situations where a structure may experience unforeseen loading conditions.
There's a growing interest in combining steel fiber reinforcement with other approaches, like utilizing conventional reinforcement bars or mesh. Initial investigations suggest that these hybrid approaches could lead to further enhanced shear strength, which may provide the best solutions for various structural applications.
As the technology related to UHPC and steel fiber reinforcement develops, new advancements are emerging in areas like automated concrete mixing processes. The goal of these developments is to create more homogeneous distributions of steel fibers throughout the UHPC mix, which, in turn, will improve the consistency of performance and improve confidence in shear strength predictions across different production batches.
Despite the clear benefits of steel fiber-reinforced UHPC for enhancing shear strength, there are still some areas needing further research. For example, the application of UHPC in shear walls is an area where only limited study currently exists. While experimental evidence suggests that shear walls constructed with UHPC exhibit favorable characteristics, further research and data are needed to define the complete design parameters and optimize their use.
Further work exploring the influence of the concrete mixture composition, fiber aspect ratio, and the use of nanomaterials combined with steel fibers would allow us to push the boundaries of what is possible for future UHPC designs for challenging shear-related structural applications. The ability to accurately predict and control the shear performance of UHPC with fibers is crucial for the widespread implementation of this innovative material, which shows great promise in areas like precast concrete-steel composite beams. By continuing research in this area, we will continue to learn more about the complex interactions and potentially reach even greater gains in the shear strength of these composites.
(As of 06 Sep 2024)
New Insights Comparative Analysis of Shear Strength in High-Performance Steel Grades for Structural Applications - Key Parameters Influencing Shear Strength in High-Performance Steel Structures
Several key factors influence the shear strength of high-performance steel structures, impacting their overall performance and integrity. The inherent mechanical properties of the high-performance steels used, including their yield strength and ductility, play a crucial role. Designers must carefully consider how to implement shear reinforcement, including the type of reinforcement, its arrangement, and spacing, to maximize the structure's shear capacity. Innovative approaches to construction, like incorporating advanced materials like ultra-high-performance concrete with steel fibers, can substantially change how shear forces are handled. Moreover, the development of sophisticated predictive models, often leveraging machine learning and finite element analysis, enables more accurate predictions of shear strength under a range of loading scenarios. These models can incorporate factors like material variability and loading rates. However, challenges remain in accurately capturing the complexities of shear behavior with these methods. As the field advances, understanding how these factors interact and refining the predictive capabilities of engineering models is essential for aligning design standards with the continuous progress in material science and construction methods, ensuring safety and structural integrity. It is critical to note that the relationship between these parameters and shear strength is often nonlinear and complex, necessitating careful experimental validation of model predictions.
Current research suggests that the internal structure of high-performance steel grades, particularly the size of its grains, plays a key role in determining its shear strength. Methods like advanced alloying are used to create finer grains, which, in turn, significantly boost shear resistance as described by the Hall-Petch relationship.
It's been observed that the temperature during steel deformation has a surprisingly strong influence on shear strength. High-performance steels show a substantial decline in shear capacity at temperatures exceeding 600°C, underlining the importance of understanding thermal behavior during design.
Modern steel production methods like thermomechanical processing create steels with exceptional characteristics exceeding those of conventional grades. However, the complexity of these transformations makes it challenging to accurately predict how the shear strength of these steels will perform in real-world applications.
Studies show that how shear reinforcement is arranged within a structure can result in a much higher shear capacity than simply increasing the amount of reinforcement. This highlights the fact that effective design shouldn't focus solely on the quantity of reinforcement, but also its specific placement and layout within the element.
The influence of the speed at which a load is applied on shear strength presents a rather unexpected trend. High-performance steels can exhibit greater shear strength under quick loading scenarios. This is an interesting counterpoint to conventional design approaches that primarily focus on static loading, and raises questions about the importance of dynamic behavior within design standards.
Advanced machine learning tools are increasingly being used to forecast the shear strength of structures made from these newer steel grades. This signifies a shift from traditional empirical methods to the use of algorithms that can predict structural performance and adapt to changes in load or other conditions for improved safety checks.
The significance of ductility, often overlooked due to the focus on yield strength, is clear in the context of shear strength. High-performance steels, under certain conditions, can fail in a brittle way. This underscores the need to carefully choose steel types based on the expected load conditions and environment.
Using a combination of conventional reinforcement materials with newer options like steel fibers and nanomaterials in high-performance concrete shows promise in maximizing shear capacity. However, the unpredictability of these interactions requires more research before it can be implemented broadly.
Interestingly, the presence of small defects or microscopic cracks within high-performance steel can negatively affect its shear strength. This highlights the importance of strict quality controls throughout production to guarantee the integrity of structures.
While predictive models for shear strength have significantly improved, current design codes do not always reflect these findings when considering high-performance steels. This means that design standards urgently need revisions to guarantee both safety and efficiency in the design of structures using modern engineering materials.
More Posts from aistructuralreview.com: