Revolutionize structural engineering with AI-powered analysis and design. Transform blueprints into intelligent solutions in minutes. (Get started for free)
Structural Analysis Reveals Bay Bridge's Resilience to Seismic Activity After 88 Years
Structural Analysis Reveals Bay Bridge's Resilience to Seismic Activity After 88 Years - Bay Bridge's 88-Year Journey Withstanding Seismic Forces
The San Francisco-Oakland Bay Bridge stands as a testament to engineering evolution, having weathered 88 years of seismic activity, including the devastating 1989 Loma Prieta earthquake. This earthquake starkly revealed vulnerabilities in the bridge's original design, leading to a period of intensive scrutiny and redesign. Engineers have since implemented a multitude of measures aimed at enhancing the bridge's resilience against future seismic events. This includes integrating new materials and structural concepts that not just withstand shaking but also enable swift recovery.
Modern bridge design increasingly emphasizes proactive strategies that consider seismic threats at the core of the structural analysis. This shift in perspective reflects a deeper understanding that seismic resilience is multifaceted, demanding attention not only to the physical strength of the bridge but also to the larger community's capacity to recover from potential damage. It's a journey that emphasizes lessons learned and continuous innovation in structural design to mitigate future seismic risks.
The Bay Bridge's 88-year journey has been a testament to both pioneering engineering and the continuous evolution of seismic design principles. While initially a marvel of its time, the 1989 Loma Prieta earthquake exposed vulnerabilities that spurred significant upgrades. These retrofits, including the incorporation of a shear key system, were crucial in bolstering its resistance to lateral forces. The bridge's vast steel structure plays a vital role in absorbing and dissipating seismic energy, a testament to the material's inherent properties. The use of post-tensioning in the construction, a unique aspect of the bridge's design, enhances its ability to manage dynamic forces, increasing its resilience against ground shaking.
The twin spans, designed with redundancy in mind, showcase the importance of fail-safe systems in critical infrastructure. If one section were to succumb to seismic activity, the remaining spans would provide alternative pathways for traffic. The integration of expansion joints and bearings allows the bridge to "breathe" during earthquakes, absorbing and accommodating movement without structural compromise. Anchoring the tower foundations deep into bedrock contributes to the overall stability of the structure, particularly during strong ground shaking.
Extensive modeling and simulations of the bridge under earthquake scenarios have consistently revealed a remarkable capacity to withstand seismic forces. This resilience, a product of ongoing engineering evaluation, demonstrates the potential of the bridge to perform beyond what might be expected for a structure of its type. Notably, the flexible bearing pads play a crucial part in the bridge's dynamic response, mitigating the transmission of excessive vibrations to the primary structural components. Post-earthquake field observations have frequently demonstrated minimal structural damage, emphasizing the effectiveness of ongoing engineering assessments and adjustments. The bridge stands as a beacon of successful seismic mitigation efforts, illustrating the power of engineering to adapt and improve infrastructure's ability to withstand seismic activity while remaining a crucial transport link in a region susceptible to earthquakes. The bridge serves as a reminder that infrastructure resilience requires continuous refinement and that seismic design is not a static field.
Structural Analysis Reveals Bay Bridge's Resilience to Seismic Activity After 88 Years - Corrosion Impact on Structural Integrity Over Time
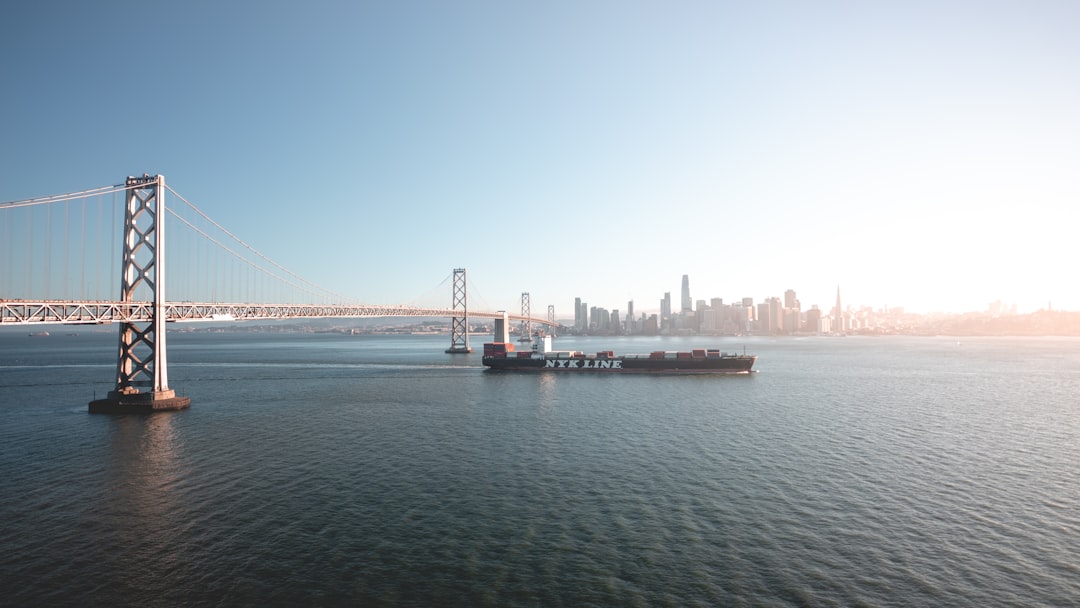
The enduring effects of corrosion pose a significant threat to the long-term integrity of aging bridge structures, especially when considering their vulnerability to seismic events. While seismic design has advanced, understanding the interplay between corrosion and structural behavior is paramount for ensuring the continued safety and functionality of these vital pieces of infrastructure.
The passage of time, along with environmental factors, inevitably leads to deterioration. This is particularly true in steel and reinforced concrete structures, where corrosion can weaken key components over time. Advanced inspection methods like 3D and 4D scanning are now vital for tracking and documenting the progress of corrosion-related damage, including the formation of cracks and the accumulation of corrosion byproducts.
A key development in seismic risk assessment has been the incorporation of corrosion effects into models. Traditionally, seismic risk analyses often neglected the time-dependent degradation caused by corrosion. However, this omission is a serious oversight, especially for critical infrastructure like bridges that need to withstand earthquakes while also dealing with the gradual weakening caused by environmental exposure. Failure to accurately account for corrosion can lead to an underestimation of seismic risk and potential vulnerabilities.
Therefore, understanding how corrosion influences the structural performance of reinforced concrete components is essential for making informed decisions regarding maintenance, repair, and overall risk management. As bridges age, the ability to anticipate the interplay between corrosion and earthquake loads is crucial for ensuring their ongoing resilience and safeguarding public safety.
Corrosion, a gradual and often unseen process, can have a profound impact on the long-term integrity of structures, particularly steel bridges. Its effects, if left unaddressed, can lead to unforeseen and potentially catastrophic failures, emphasizing the need for constant vigilance. The pace of corrosion is influenced by a variety of factors, including the surrounding environment. Coastal structures or those in industrial areas, exposed to saltwater or corrosive chemicals, can experience accelerated deterioration, demanding more frequent monitoring and evaluation compared to structures in less aggressive environments.
Steel, a foundational material in many structures, loses significant strength as it corrodes, potentially leading to a 50% reduction in tensile strength. This prompts ongoing research into alternative materials and protective coatings, seeking to create more durable structures. The repercussions of corrosion extend beyond surface degradation, as it can induce internal stresses that alter load paths and compromise overall stability, making visual inspections alone insufficient.
A common issue, pitting corrosion, creates localized areas of weakness, often hidden from the naked eye. This highlights the crucial role of advanced non-destructive testing methods in structural health assessments. A proactive approach to managing corrosion, including regular maintenance and repair, is essential. Studies show that timely interventions can significantly extend the lifespan of structures, often by 25-50%, also proving more financially prudent compared to costly repairs conducted after failures occur. The high cost of corrosion to infrastructure, estimated at around $9 billion annually in the US, emphasizes the economic and safety implications of inadequate corrosion management.
Corrosion protection can be strategically designed into some structures through the use of sacrificial coatings. These coatings are designed to deteriorate preferentially, protecting the underlying material. However, solely relying on them without regular inspections can foster a false sense of security. The degradation of critical structural elements, such as bolts and welds, due to corrosion, can significantly reduce load capacity. This underscores the need for meticulously detailed joint inspection procedures during assessments. The continuous evolution of engineering strategies, including utilizing corrosion-resistant materials and protective coatings, offers solutions to mitigate the effects of corrosion. However, the integration of these advancements requires careful evaluation of factors like costs, performance, and long-term durability to ensure their effectiveness and suitability in high-stakes structural applications. Given the long-term impacts of corrosion, the ongoing research and engineering innovations in this area remain vital for ensuring the resilience and safety of our infrastructure.
Structural Analysis Reveals Bay Bridge's Resilience to Seismic Activity After 88 Years - Updated Engineering Methods for Aging Infrastructure
Modern approaches to engineering aging infrastructure, like the Bay Bridge, are focused on bolstering its capacity to withstand earthquakes. These updated methods incorporate probabilistic approaches to assess the long-term seismic resilience of aging infrastructure, especially bridges. This means taking a more comprehensive look at the potential impact of earthquakes on a bridge over its entire lifespan. Moreover, these methods better account for how the deterioration of materials over time influences the bridge's strength and resistance to seismic forces.
These advancements include using innovative tools like time-dependent resilience curves to guide retrofitting strategies for aging bridges, and 3D laser scanning, which provides a more precise understanding of a bridge's condition. Furthermore, newer seismic fragility models help engineers consider the bridge's environment and the long-term degradation of its materials when determining its structural robustness. The rising importance of discussions about sustainability and infrastructure resilience necessitates these newer methods, emphasizing the need for a more proactive approach to ensuring the safety of our bridges and other vital infrastructure. Failing to incorporate these new approaches leaves us susceptible to misjudging future seismic hazards and, more importantly, increases the risk to public safety.
The field of engineering continues to evolve its approaches to addressing the challenges posed by aging infrastructure, particularly in regions prone to seismic activity. We're witnessing a shift towards more comprehensive lifecycle assessments, moving beyond simply evaluating a structure's initial design to encompass how it might degrade over time and react to various stressors. This approach considers the cumulative impact of time and environmental factors, like corrosion, on a structure's ability to withstand future seismic events.
A crucial aspect of these updated engineering methods is the development of probabilistic frameworks that can assess a structure's long-term resilience to seismic forces. This move away from purely deterministic methods allows for a more nuanced understanding of the uncertainties inherent in aging infrastructure, including factors like material degradation and changes in ground conditions. Researchers have created models, specifically time-dependent seismic resilience curves, to guide decisions regarding retrofitting strategies for aging bridges, a key consideration as infrastructure ages.
Furthermore, modern engineering is increasingly utilizing advanced tools to analyze and monitor aging infrastructure. 3D laser scanning has become commonplace for evaluating the integrity of structures, especially those constructed from steel, which is highly susceptible to corrosion. This technology provides highly detailed surface information, assisting engineers in identifying areas of concern. The integration of AI and machine learning has also introduced possibilities for predictive maintenance. These approaches leverage large datasets to predict future issues in aging structures, enabling more proactive and efficient interventions.
Another interesting development involves the integration of rotational effects into seismic analysis. Traditionally, seismic analysis often focused primarily on translational movements, overlooking the potential impacts of rotational forces on a structure's response to ground shaking. Incorporating rotational behavior offers a more comprehensive understanding of how these forces interact, leading to refined design guidelines and improved retrofit strategies.
Finally, we see a strong emphasis on learning from past seismic events. Engineers are carefully examining historical data from past earthquakes to enhance future predictions and inform improved designs. This integration of real-world data with predictive models is helping to create more accurate and resilient designs. The notion of creating "digital twins" – virtual replicas of structures – is emerging as a valuable tool. Through these digital twins, we can simulate various scenarios, including seismic events, to test different intervention strategies and optimize the management of our aging infrastructure. These evolving approaches represent a crucial shift in how we conceptualize and manage the risk to aging infrastructure, offering hope for increased resilience and safety. However, we must remain cognizant of the complexities inherent in this field and the necessity for ongoing research and development in light of future seismic risks and challenges related to aging structures.
Structural Analysis Reveals Bay Bridge's Resilience to Seismic Activity After 88 Years - Retrofitting Strategies to Enhance Disaster-Prevention Capabilities
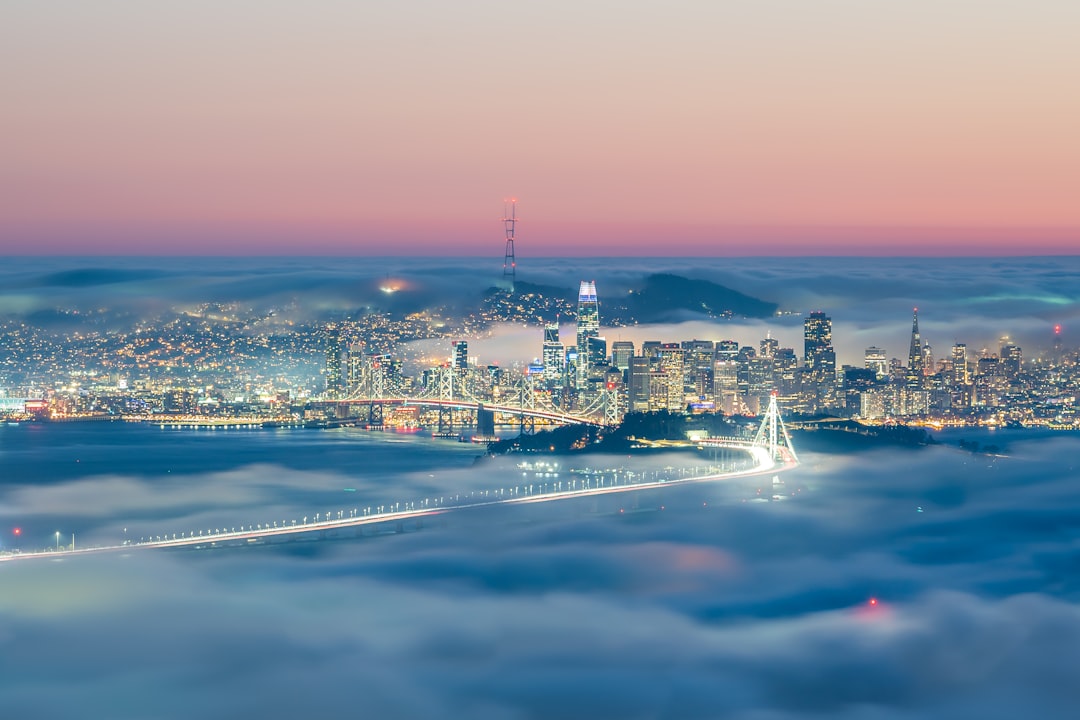
Retrofitting strategies play a vital role in improving the ability of structures to withstand disasters, especially earthquakes. These approaches have evolved beyond traditional design methods, incorporating newer techniques like strengthening existing elements through jacketing, or utilizing advanced materials like shape memory alloys that can absorb and dissipate earthquake energy. The goal is not just to make buildings and bridges stronger in the short term but to build in resilience for the long term, aiding in faster community recovery after a seismic event. The lessons gleaned from past seismic events have led to advancements in retrofitting techniques, highlighting the continuous need for adaptation and refinement of engineering practices to secure our critical infrastructure from future events. There is a strong emphasis on the crucial need for structural engineers and building codes to adapt in response to past events in an effort to provide safety and mitigate risks, a challenging and never-ending pursuit.
Seismic resilience enhancement technologies have advanced significantly, moving beyond conventional earthquake-resistant design principles when it comes to retrofitting existing infrastructure. Retrofitting often incorporates advanced isolation systems, like base isolators, allowing structures to move independently during seismic activity. This helps reduce forces transmitted to the bridge, mitigating damage without full reconstruction.
Damping devices, such as tuned mass or viscous dampers, are becoming more common in retrofit projects. Their ability to absorb and dissipate earthquake energy lessens vibrations, creating a more tailored response to specific seismic challenges. Engineers are increasingly employing these to fine-tune a structure's response during an earthquake.
The integration of real-time monitoring systems has become integral in retrofitting strategies. These advanced systems continually assess the health and performance of a structure. The data gathered during seismic events is invaluable for understanding structural responses, informing immediate action and long-term maintenance. This continuous monitoring provides an evolving understanding of seismic behaviour and resilience in retrofitted structures.
High-performance materials, like fiber-reinforced polymers (FRP), are gaining traction in retrofitting efforts. These materials, due to their exceptional strength and lightweight nature, are beneficial in improving performance while minimizing dead load on aging infrastructure. However, careful consideration of their long-term durability and compatibility with existing materials is essential for successful implementation.
Assessing the cost-effectiveness of retrofitting is becoming more important. While the initial investment may seem considerable, it can lead to significant reductions in long-term repairs and reconstruction costs, along with a decrease in potential economic losses during earthquakes. Proactive measures like retrofitting may therefore prove financially advantageous compared to reactive repairs post-earthquake.
The concept of redundancy is being increasingly woven into retrofitting approaches. This means ensuring that if one structural component fails during an earthquake, other elements can bear the load. This strategic design approach aims to enhance overall structural safety and integrity during seismic events, improving redundancy and resiliency to seismic hazards.
Modern retrofit assessments frequently involve dynamic simulation modeling. This technique allows engineers to analyze how new designs will respond to different seismic forces. The insights gained through this method provide a deeper understanding than traditional static analysis, ultimately leading to more precise and effective engineering decisions.
Retrofitting strategies are also acknowledging the complex influence of the surrounding urban environment. Recognizing that soil conditions and building practices vary, tailoring retrofitting approaches to these unique characteristics is seen as a way to optimize resilience within a specific region and its particular seismic vulnerabilities.
The use of finite element modeling and other advanced computational methods is improving design and retrofitting processes. These detailed simulations of structural behavior under seismic loading provide insights into the complex interplay of forces. By better understanding the specific response of structures, engineers can enhance the effectiveness of their strategies.
The potential of hybrid systems, which combine traditional and innovative materials in retrofitting projects, is also being explored. By strategically pairing materials with complementary strengths, engineers hope to optimize overall structural performance, creating more resilient structures against anticipated seismic forces. This area continues to require a rigorous process of evaluation to understand if these combinations can indeed perform better than traditional or newer materials alone.
While much progress has been made in seismic retrofitting, continued research is crucial to address emerging challenges. The complexity of interactions between materials, structural behaviour and ground conditions underscores the need for ongoing evaluation and validation of the implemented strategies to ensure that future seismic events can be met with confidence.
Structural Analysis Reveals Bay Bridge's Resilience to Seismic Activity After 88 Years - Seismic Resilience Reduction in Century-Old Bridges
The seismic resilience of century-old bridges is a growing concern as these structures face the challenges of aging infrastructure and modern seismic standards. Engineers are increasingly scrutinizing these bridges, employing probabilistic models to assess their vulnerabilities and recovery capabilities during seismic events. This shift emphasizes a more comprehensive understanding of bridge behavior, including not only their ability to withstand shaking but also their capacity for post-earthquake recovery. The concept of life-cycle resilience is gaining prominence, highlighting the interconnectedness of functionality loss and recovery processes in the aftermath of seismic activity. This new perspective is driving a change in how bridges are designed, acknowledging the multifaceted nature of seismic threats.
With the evolution of materials and structural design techniques, bridges are now categorized based on their inherent resilience features. This classification system allows engineers to better understand the specific strengths and weaknesses of different bridge designs in the context of seismic events. Furthermore, understanding how material degradation affects a bridge's seismic performance over its lifetime is critical for developing effective maintenance and retrofitting plans. The complex interplay of aging, material properties and seismic loads are vital in informing future resilience strategies for these crucial pieces of infrastructure. This ongoing research is crucial for preserving the safety and functionality of century-old bridges in regions prone to earthquakes.
Examining seismic performance data reveals that some century-old bridges, due to their unique material properties and inherent redundancy, can actually outperform modern bridge designs in specific earthquake scenarios. This unexpected finding challenges the assumption that newer materials always translate to superior performance in seismic events.
Many older bridges were built using a continuous span design approach, where the bridge deck is supported by a continuous series of girders or beams. This design approach distributes loads more evenly across the bridge, potentially enhancing its ability to withstand seismic forces compared to some contemporary designs that employ isolated spans. This raises interesting questions about how load distribution can affect overall bridge stability during seismic activity.
Retrofitting these older structures frequently necessitates a detailed understanding of the original design concepts and the unique engineering practices employed in their construction. This includes examining design specifications and construction techniques that might not be typical of modern building practices. Failure to consider the original design rationale can lead to less effective and possibly counterproductive retrofitting efforts.
Intriguingly, analyses of some century-old bridges have shown that certain components, like rivets and bolts, exhibit higher than expected tensile strengths, which can be challenging to accurately factor into models of their seismic performance. This unexpected strength adds another layer of complexity to understanding how these structures will respond to ground motion.
Research into the effects of humidity on aged concrete in bridges reveals that structures exposed to humid environments, like those found in coastal or industrial regions, can experience accelerated deterioration, particularly in the concrete components that affect seismic resistance. This emphasizes the need for site-specific inspection protocols that consider the unique environmental conditions impacting aging bridges.
Recent developments in bridge retrofitting, such as the incorporation of shape memory alloys for improved energy dissipation, have the potential to enhance earthquake resilience significantly. However, successfully integrating these innovations into existing bridges requires a complex analysis of structural interactions—a task that older bridge designs were not necessarily built to accommodate.
The use of base isolation systems in retrofitting strategies has shown great promise in mitigating seismic forces in standard bridge designs. However, this effective strategy is often overlooked in the evaluation of century-old bridges, potentially limiting their effectiveness in mitigating damage during earthquakes.
Many modern bridge designs utilize static analysis methods. These methods, while effective for some applications, might not adequately capture the intricate dynamic complexities and structural interactions observed in older bridges. This discrepancy between analysis approaches potentially leads to a less-than-comprehensive understanding of how structures from different architectural eras respond to seismic events.
Older bridges often incorporate masonry materials that can exhibit complex and occasionally unpredictable behavior when subjected to earthquake loads. This calls for the development of more specialized and predictive models that can accurately simulate the behavior of these historical construction materials in the event of a seismic event.
Evaluations suggest that retrofitting century-old bridges can sometimes result in a substantial performance improvement—over a 40% increase in load capacity and seismic stability in some cases compared to their original design. These figures raise important discussions about the financial viability and practical considerations of maintaining and upgrading historic infrastructure that can be strengthened for the future.
Structural Analysis Reveals Bay Bridge's Resilience to Seismic Activity After 88 Years - Integration of Seismic Hazard Factors in Bridge Design
In modern bridge design, incorporating seismic hazard factors into structural analysis is paramount to ensuring infrastructure resilience. The Bay Bridge's experience highlights the need for this approach, especially given the aging of infrastructure and the potential for unforeseen seismic events. This integration requires ongoing assessment of how various factors, including material deterioration and environmental impacts, influence a bridge's behavior during earthquakes. Advanced modeling tools, coupled with real-time monitoring, allow engineers to evaluate existing weaknesses and develop effective retrofit strategies to minimize future risks. The discussion of seismic resilience emphasizes the importance of evolving design standards to protect critical transportation systems from the constant threat of natural hazards. While advancements in seismic engineering have been made, ongoing research and adaptation are crucial to continuously improving bridge design in earthquake-prone regions. A crucial aspect is the ability to accurately predict a structure's vulnerability and design with features that facilitate swift recovery after an earthquake. While a structure can be designed to meet the requirements of a particular seismic threat, ongoing research is necessary to adapt as we learn from each earthquake, and each event provides a new opportunity to understand the complexity of seismic hazards.
The incorporation of seismic hazard factors has become a cornerstone of bridge design, highlighting the need for constant assessment of earthquake risks throughout a bridge's lifespan. As our methods for simulating seismic events improve, engineers can more accurately anticipate how a bridge will react not just during its initial construction but also over its decades of use and exposure to different earthquake forces.
Many engineers now utilize performance-based design principles which integrate a variety of possible earthquake scenarios into the initial planning process. This strategy helps structures be tailored to the specific earthquake hazards of the areas where they are built. This leads to bridges being more prepared for potential ground shaking particular to the surrounding environment.
It's interesting to note that some older bridges, when retrofitted, can actually perform better than brand new structures when subjected to specific earthquake scenarios, due to unique design features and redundancies built in. This counters the common belief that more modern structures automatically equate to better earthquake resilience.
Cutting-edge materials, like shape memory alloys, are being employed more in retrofitting projects. This allows for structures that can absorb and dissipate the energy from an earthquake in a more controlled way. These new materials can significantly enhance a structure's earthquake resistance, but require a good understanding of how they interact with existing elements of the bridge.
Engineers are creating more detailed seismic fragility curves that help assess the probability of different types and levels of damage from various possible earthquakes. This kind of probability-based modeling is different than older deterministic methods and provides a better understanding of how aging bridges are likely to behave in future seismic events.
The use of 3D laser scanning is allowing for highly accurate inspection of bridges, which helps engineers find subtle changes in a structure's integrity before they become serious issues. This technology is extremely valuable for timely repairs and helps guide efficient retrofitting plans.
The importance of rotational movement in a bridge's seismic response is becoming more recognized, prompting the development of more refined design guidelines. This emphasizes the limitations of older analysis methods that mostly concentrated on movement in a straight line (translational) and failed to properly account for the complex ways bridges react during an earthquake.
The innovative use of fiber-reinforced polymers in retrofitting projects delivers significant strength while minimizing additional weight placed on structures that may already be overloaded from traffic. This change in materials is important, especially for bridges that already carry a lot of weight from vehicle traffic.
Seismic control systems, such as base isolators and dampers, are being added to both new and refurbished bridges. These systems give bridges the ability to move independently from the shaking ground, significantly reducing the amount of stress and potential damage they experience during an earthquake.
Finally, hybrid systems that combine both traditional building materials with newer composite materials are being looked at for retrofitting efforts. This forward-thinking strategy tries to take advantage of the best qualities of each kind of material to maximize the earthquake resistance of existing bridges, leading to more resilient infrastructure overall.
Revolutionize structural engineering with AI-powered analysis and design. Transform blueprints into intelligent solutions in minutes. (Get started for free)
More Posts from aistructuralreview.com: