Revolutionize structural engineering with AI-powered analysis and design. Transform blueprints into intelligent solutions in minutes. (Get started for free)
The Evolution of Integrated Circuits From Transistors to Billion-Component Microchips in 2024
The Evolution of Integrated Circuits From Transistors to Billion-Component Microchips in 2024 - From Kilby's Hybrid to Noyce's Monolithic The Birth of Integrated Circuits
The journey from Jack Kilby's initial hybrid integrated circuit concept to Robert Noyce's monolithic approach represented a significant leap forward in electronics. Kilby's 1958 invention paved the way, but Noyce's innovation of integrating all circuit elements onto a single semiconductor crystal truly unlocked the potential of this technology. This paradigm shift, with its promise of greater efficiency and performance, began to take hold in the 1960s, fueled by applications in both military and consumer products. Fairchild Semiconductor's introduction of commercially available integrated circuits further solidified this burgeoning field. Noyce's pioneering work simplified design complexities and opened the door to more intricate and powerful electronic devices. This advancement ultimately led to the development of the microprocessor, a cornerstone of modern computing, which highlights the transformative impact of integrated circuits. The evolution of integrated circuits has been characterized by a steady increase in both complexity and miniaturization, a trend that has profoundly influenced the course of electronic innovation for generations.
Jack Kilby's initial foray into integrated circuits in 1958 was a breakthrough, demonstrating the potential of combining multiple circuit elements into a single package. His approach, though innovative, used germanium and was classified as a hybrid circuit—meaning it combined discrete components on a substrate. This early work, while significant, laid the groundwork for a more impactful approach.
In 1959, Robert Noyce's concept of the monolithic integrated circuit emerged as a significant improvement. This approach, integrating all components onto a single piece of silicon using a planar process, offered superior control over electrical characteristics. This meticulous control of the semiconductor led to enhanced device performance and paved the way for the future of microelectronics.
Both Kilby and Noyce's groundbreaking work arose from a critical need in the 1950s, a period of escalating demand for smaller, more energy-efficient electronic devices for military and communications purposes. The desire to meet these needs spurred them to find better ways to design and fabricate electronic devices.
It's fascinating to note that while both were pioneers in the field, their paths diverged slightly regarding the commercial and intellectual property aspects. Kilby received the first integrated circuit patent, while Noyce's subsequent contributions focused on refining manufacturing processes. Noyce's efforts, in turn, were essential to the advancement of semiconductor scaling, a cornerstone of modern microchip design.
The dynamic competition created by these inventions propelled the rapid evolution of semiconductor technology. The industry shifted from cumbersome and expensive vacuum tubes to more compact and affordable semiconductor solutions. This transition, driven by the ingenuity of these early innovators, changed consumer electronics forever.
This fundamental change impacted much more than just how we consume electronics. Integrated circuits enabled advances in digital logic, microprocessors, and memory, leading to what we now call the microelectronics revolution. This revolution continues to shape and propel modern computing and countless related technologies.
Furthermore, Noyce's impact wasn't limited to circuit design. He co-founded Intel, a company that would later play a central role in shaping the future of personal computers by focusing on the microprocessor. This focus further revolutionized computer architecture and gave birth to the era of widespread computing.
The principles established by both Kilby and Noyce, even today, remain foundational for modern chip design. This is true across the spectrum of microelectronics, from basic RFID tags to advanced microcontrollers found in sophisticated modern devices.
The influence of the integrated circuit reaches far beyond electronics. Industries like automotive, aerospace, and telecommunications have benefited profoundly from this invention, highlighting the broad reach of a single, ingenious innovation. It truly serves as a testament to the power of innovative thinking and its impact on shaping the world around us.
The Evolution of Integrated Circuits From Transistors to Billion-Component Microchips in 2024 - Moore's Law The Driving Force Behind Transistor Density Growth
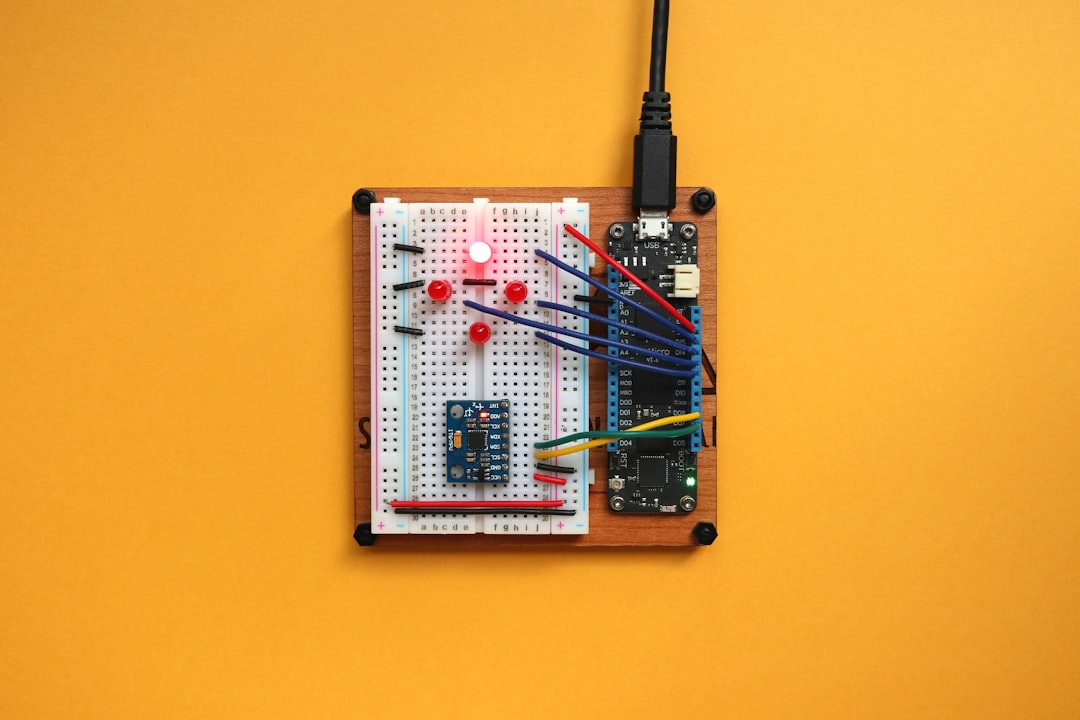
Gordon Moore's 1965 observation, now known as Moore's Law, proposed that the number of transistors integrated onto a chip would roughly double every two years. This prediction, more of a trend than a physical law, has been a powerful driver of advancements in computing. It's reflected in the consistent growth of transistor density, a core factor in improving chip performance and efficiency.
For over 50 years, this doubling trend has held remarkably true, with the average microprocessor transistor count increasing exponentially, from just over 2,000 in 1971 to a staggering 582 billion by 2021. This incredible growth is intertwined with both the increasing number of transistors on a chip and the simultaneous miniaturization of each individual transistor.
The continuous development of manufacturing processes, particularly in the realm of CMOS technology, has allowed for ever-smaller transistors, currently measured in nanometers. While Moore's Law continues to offer a valuable framework for understanding the trajectory of integrated circuits, we are now entering an era where the physical limitations of miniaturization become more pronounced. It remains to be seen if the predicted doubling rate can be sustained in the coming years, as the industry faces new obstacles in pushing the boundaries of chip density further. The future of Moore's Law, once considered a sure bet, is now a matter of debate and exploration.
Gordon Moore's 1965 observation, now known as Moore's Law, suggested that the number of transistors on a microchip would roughly double every two years. This has held remarkably steady for a considerable time, shaping how the technology industry has approached development and projected growth. The drive to shrink transistors has resulted in chips with incredibly small features, down to just a few nanometers, allowing billions of transistors to reside on a single piece of silicon. This has, of course, dramatically improved computing power while simultaneously minimizing the energy needed to run these devices.
However, maintaining this trend has become more challenging lately. As transistors approach atomic scales, quantum tunneling effects start to become a problem, potentially jeopardizing the viability of traditional silicon-based chips. It's important to remember that "Moore's Law" isn't a fundamental law of physics, but rather an observation about the fast-moving evolution of semiconductor design and fabrication. It's not a guarantee, but rather a reflection of trends.
Intel's 4004, the first commercially available microprocessor, debuted in 1971, demonstrating the potential of Moore's vision by squeezing 2,300 transistors onto a single chip. This early milestone highlighted how integrated circuits could revolutionize the field of computing. While transistor count is significant, Moore's Law isn't solely focused on this one metric. Improvements in performance, coupled with dramatic reductions in the cost per transistor, have made cutting-edge technology more widely available.
There's a growing discussion among researchers that the traditional scaling predicted by Moore's Law might be reaching its end. This has fueled exploration into new computing paradigms like quantum computing and neuromorphic architectures, which could potentially redefine our understanding of future computational boundaries. The relentless pursuit of smaller transistors has necessitated huge investments in the photolithography equipment required to create the complex patterns used in these high-density chip designs. These manufacturing facilities cost billions of dollars to build, representing a substantial financial commitment by the industry.
The race to extend Moore's Law has also led to significant mergers and acquisitions in the tech industry. Companies are seeking to pool resources and expertise to push the boundaries of silicon manufacturing. Recently, there has been increasing attention on the potential of materials science to potentially provide new paths to extend the growth projected by Moore's Law. Research into graphene and other 2D materials may provide solutions to overcome some of the limitations of traditional silicon technology. While Moore's Law's future path may be unclear, it's certain that the drive to miniaturize and improve integrated circuits will continue to be a significant driving force in the technology industry.
The Evolution of Integrated Circuits From Transistors to Billion-Component Microchips in 2024 - Nanoscale Fabrication Techniques Enabling Billion-Component Chips
The creation of microchips containing billions of components is now possible thanks to advancements in nanoscale fabrication techniques. This represents a critical stage in the progression of semiconductor technology. The ongoing drive for higher transistor density within chips has led to the exploration of alternative materials, such as two-dimensional materials and semiconductor nanowires, due to the limitations of traditional silicon-based methods. These innovative materials, alongside the development of sophisticated frontend-of-line (FEOL) and backend-of-line (BEOL) processing techniques, are fundamental to the continuing pursuit of miniaturization and enhanced performance. These enhancements are essential for emerging technologies that demand greater computational power, including the fields of big data, artificial intelligence, and the Internet of Things. Yet, as the industry grapples with challenges stemming from physical constraints and the mounting costs of advanced fabrication, a re-evaluation of traditional approaches is becoming increasingly necessary. This search for new solutions is vital. The journey towards billion-component chips is a remarkable achievement, but it also serves as a reminder of the ever-growing complexity and challenges present in designing modern integrated circuits.
Creating chips with billions of components necessitates incredibly precise fabrication techniques at the nanoscale. Techniques like extreme ultraviolet (EUV) lithography are crucial for etching features smaller than 10 nanometers, a key factor in pushing transistor density further. This technology has been pivotal in extending the trend of shrinking transistor sizes.
Atomic layer deposition (ALD) has become a critical part of this process. ALD allows for the precise layering of materials, one atom at a time. This level of control is essential for creating high-quality gate oxides and other components in modern transistors, enhancing performance and reliability.
While traditional photolithography has been dominant, alternative approaches like nanoimprint lithography are gaining attention. These nanostructuring techniques hold promise for lowering the cost of chip production while still achieving the incredibly high resolutions needed for billion-component chips.
Self-assembly methods, which utilize the ability of certain materials to spontaneously organize into specific patterns, are also being explored. These techniques could potentially revolutionize chip fabrication by streamlining the integration of components onto the chip, potentially reducing manufacturing complexities.
The use of novel materials, especially two-dimensional (2D) materials like graphene, is becoming increasingly important. These materials exhibit excellent electrical properties compared to traditional silicon, leading to potential performance gains and reduced energy consumption in future microchips.
Quantum dots, which are incredibly small semiconductor nanocrystals, are another area of intense research. Their unique electronic characteristics offer the possibility of more efficient charge transport, which could potentially lead to smaller and more powerful electronic components within the chips.
However, as we continue to push the boundaries of miniaturization, we're facing new challenges rooted in the principles of quantum mechanics. Phenomena like quantum tunneling, where electrons can 'leak' through barriers, become more prominent at the nanoscale and can significantly impact transistor performance. Researchers are working to address these issues by exploring alternative computing approaches, such as spintronics.
The design and manufacturing process itself is also evolving, with machine learning and AI being increasingly integrated. These technologies allow for more effective optimization of chip layouts and a reduction in errors during fabrication, improving yield and quality.
The inherent limitations of traditional physics are becoming increasingly apparent at the nanoscale. This forces engineers to reconsider traditional design approaches, leading to exploration of unconventional computing paradigms. These new paradigms could potentially reshape how chips operate and potentially surpass the capabilities of current technology.
There's a growing discussion about the fundamental physical limits of miniaturization. Some experts believe that future innovation may not solely rely on continued shrinking of transistor sizes but instead on fundamentally different architectures and approaches. This could potentially lead to a radical shift in how we design and fabricate microchips in the future.
The Evolution of Integrated Circuits From Transistors to Billion-Component Microchips in 2024 - Beyond Silicon Exploring Novel Materials for Next-Gen ICs
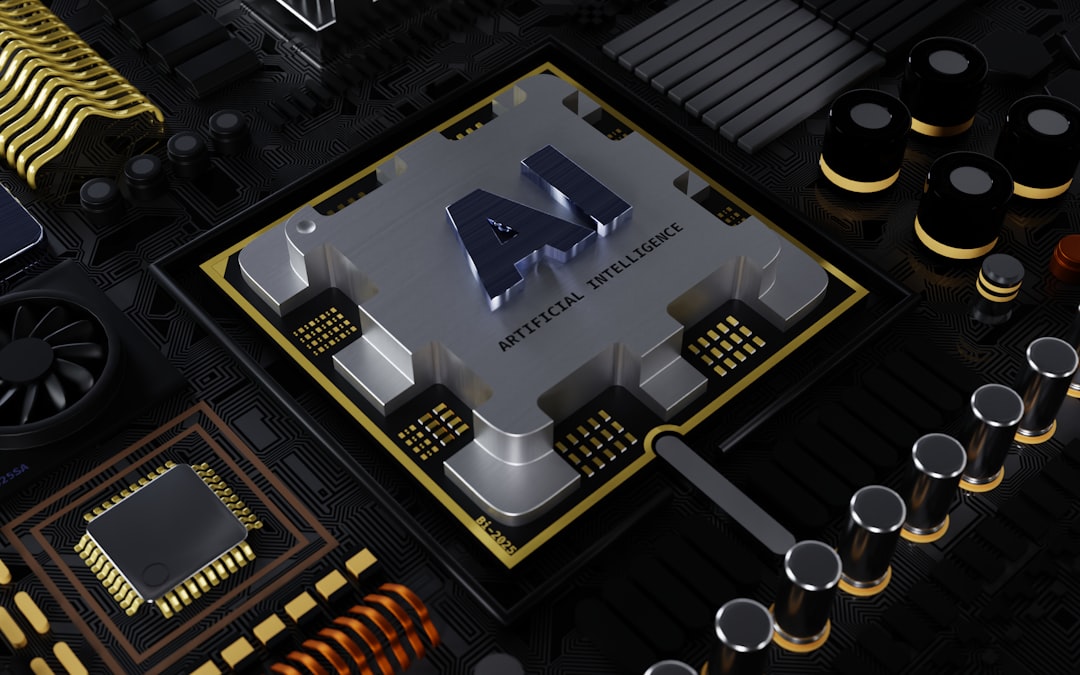
The relentless pursuit of smaller, faster, and more efficient integrated circuits has pushed the boundaries of silicon technology to its limits. As Moore's Law faces growing challenges in sustaining its historical trend of transistor density growth, the semiconductor industry is actively exploring novel materials to propel the development of next-generation integrated circuits. Two-dimensional (2D) materials, such as graphene and transition metal dichalcogenides (TMDs), are emerging as potential replacements for silicon, offering enticing electrical characteristics that could potentially revolutionize chip design and performance. The atomic-scale thickness of these materials allows for the creation of transistors at dimensions below 1nm, overcoming some of the limitations that plague current silicon-based designs. These advancements could lead to dramatic increases in transistor density within a given chip area, paving the way for smaller, faster, and more power-efficient electronics. However, incorporating these novel materials into existing manufacturing processes presents considerable challenges. Moreover, the transition to sub-1nm feature sizes introduces new complexities associated with quantum mechanical effects, potentially impacting the reliability and stability of such devices. The future of integrated circuits rests on successfully addressing these challenges, fostering innovation, and navigating the intricate landscape of materials science to fulfill the promise of this next technological wave.
The journey towards ever-more-powerful integrated circuits (ICs) is pushing the boundaries of silicon's capabilities. Silicon, the workhorse of electronics for decades, is facing fundamental constraints in scaling down further. This has spurred a surge of research into a diverse array of novel materials.
Two-dimensional (2D) materials, like graphene and transition metal dichalcogenides (TMDs), are gaining traction. Graphene, a single atomic layer of carbon atoms, possesses exceptional electrical conductivity and speed, potentially leading to far faster transistors. TMDs, including molybdenum disulfide (MoS₂) and tungsten diselenide (WSe₂), offer enhanced electrostatic control and thinner structures, which can boost nanoscale device performance.
Beyond these 2D materials, topological insulators are proving to be fascinating. Their unique electrical properties, conducting electricity on the surface while remaining insulating within, could enable faster electronic components and spintronic devices. The field of spintronics aims to leverage electron spin for data processing, potentially leading to more efficient computation.
The applications of quantum dots extend beyond the realm of transistors and are finding niches in displays and solar cells. Their ability to precisely control charge could result in more efficient transistors within future generations of electronics.
The current trend of 2D chip scaling might soon see a shift towards three-dimensional (3D) architectures. 3D ICs enable vertical stacking of components, easing the congestion of planar designs and enabling significantly higher transistor densities.
Strain engineering has demonstrated the potential of applying stress to silicon to enhance its electrical properties. This results in faster electron mobility and high-performance transistors.
Spintronics, as a field, suggests that leveraging electron spin along with their charge could yield faster and more energy-efficient devices. This could be particularly relevant in creating computation that reduces heat generation.
The discovery and application of new ferroelectric materials could potentially lead to semiconductor devices with lower voltage requirements. These materials use electric polarization for charge transport, offering an energy-efficient approach to integrated circuits.
Neuromorphic computing, a radical departure from traditional von Neumann architectures, mirrors the human brain's structure and function. This approach could be a significant advancement in handling complex datasets, offering great potential in the domain of artificial intelligence.
The need for alternative materials and approaches suggests that Moore's Law, in its traditional form based on silicon scaling, may be reaching its physical limits. It's likely that innovation, possibly utilizing the concepts behind quantum computing or novel molecular-scale devices, will be needed to push integrated circuit capabilities to the next level. The future of electronics seems bright with the exploration of novel materials beyond silicon, although these new directions present their own sets of challenges and uncertainties.
The Evolution of Integrated Circuits From Transistors to Billion-Component Microchips in 2024 - The Slowing of Moore's Law Challenges and Solutions in 2024
The continued miniaturization of transistors, a cornerstone of Moore's Law, is facing increasing challenges in 2024. The relentless pursuit of smaller transistors, while delivering incredible increases in chip performance, is now encountering fundamental physical limitations. Issues like quantum tunneling and the complexities of fabricating ever-smaller features are making it difficult to maintain the historical trend of doubling transistor density every two years. This has spurred a search for new solutions, including exploring the potential of two-dimensional materials, which offer the intriguing possibility of transistor dimensions below the atomic level. Furthermore, the landscape of chip architecture is evolving with novel designs that could potentially overcome the limitations of traditional silicon-based approaches. The growing global competition in the semiconductor sector has also led to government initiatives, like the CHIPS Act, aimed at stimulating domestic research and fostering technological advancements. The industry's path forward will likely involve embracing new materials, pushing the boundaries of chip design, and a continued commitment to innovation in the face of complex technological hurdles. The future of Moore's Law, once a seemingly predictable trajectory, is now a dynamic space demanding adaptation and creativity.
Gordon Moore's 1965 observation, now known as Moore's Law, predicted that the number of transistors on a chip would roughly double every two years. While this trend held strong for decades, recent projections suggest a slowdown, with transistor density now predicted to increase by about 1.5 times every two to three years. This shift raises serious questions about the continued viability of traditional scaling approaches in the semiconductor industry.
One of the most pressing challenges facing the industry is managing heat dissipation. As we cram more and more transistors onto chips, power density increases significantly. This necessitates sophisticated and innovative cooling solutions to prevent the chips from overheating, which can lead to failures and performance degradation.
At the nanoscale, quantum effects, specifically quantum tunneling, become increasingly pronounced. Electrons can 'tunnel' through barriers that they would normally be blocked by, potentially leading to signal integrity problems in chips with transistors measured in just a few nanometers.
The continued miniaturization of transistor features has relied heavily on advanced lithography techniques, particularly extreme ultraviolet (EUV) lithography. However, the cost of EUV equipment, which can easily reach $150 million per machine, has a substantial impact on chip manufacturing economics. This cost factor heavily influences investment strategies and manufacturing capacity decisions.
Recognizing the limitations of silicon alone, researchers are actively investigating hybrid approaches that combine silicon with novel materials like graphene or TMDs. The goal is to leverage the advantages of both while overcoming the limitations of either individually.
The field of 2D materials is showing considerable promise. These materials, only a single atom or molecule thick, appear to be capable of achieving transistor performance levels that silicon can't reach, particularly regarding speed and energy efficiency. This holds exciting possibilities for future microchip designs.
The physical constraints associated with shrinking transistor sizes have spurred exploration of 3D integrated circuit architectures. These 3D chips can stack transistors vertically, enabling a considerable increase in transistor density without requiring smaller individual transistors. This could help extend the benefits of Moore's Law beyond the current projected growth rates.
Neuromorphic computing offers a drastically different approach to computation, inspired by the human brain's neural structure. Rather than the linear and sequential processing seen in conventional computer architectures, neuromorphic computing operates in a more parallel and adaptable way. This paradigm shift could potentially bypass some of the challenges facing scalar transistor growth.
Materials science has become increasingly important in the semiconductor industry. For example, strain engineering has demonstrated the potential to improve silicon performance by altering the physical properties of the material through stress. This can increase electron mobility in existing silicon-based technologies.
The approaching physical limits of Moore's Law are prompting deep exploration into fields like quantum computing and spintronics. These technologies leverage fundamentally different principles to process data and might require a substantial shift in how we think about computation going forward. The near future of computation may require a fresh perspective, going beyond the traditional approaches that have served us well until now.
The Evolution of Integrated Circuits From Transistors to Billion-Component Microchips in 2024 - Societal Impact How Integrated Circuits Shaped the Digital Age
Integrated circuits have fundamentally reshaped the digital age, profoundly impacting nearly every facet of modern life. Their ability to condense numerous electronic components onto small chips has driven significant improvements in computing capabilities, energy efficiency, and the accessibility of complex technologies. This technological surge has sparked innovation in numerous fields – including telecommunications, healthcare, and transportation – revolutionizing information processing and communication.
However, as the 21st century unfolds, the limitations of relentless miniaturization and challenges inherent in conventional silicon-based technologies necessitate a critical reevaluation of the future direction of integrated circuits and their broader societal impact. This ongoing evolution raises fundamental concerns about the long-term sustainability and the ethical implications of our increasing reliance on sophisticated and ever-more-complex electronic systems. It remains to be seen how we'll navigate this evolving landscape and manage the complex interplay of innovation and societal impact.
Integrated circuits, those minuscule chips no larger than a fingernail, have fundamentally reshaped technology and society. Their compact size and efficiency replaced bulky, power-hungry components, leading to a revolution in electronics. The journey started with Jack Kilby's initial hybrid concept in 1958 and saw a pivotal leap forward with Robert Noyce's monolithic approach, where all circuit components were integrated onto a single crystal. This innovative approach, first commercialized by Fairchild Semiconductor, laid the groundwork for future advancements.
The complexity of integrated circuits has grown immensely over time, with transistor counts on a single chip increasing from a few thousand in the early 1970s to billions in 2024. This relentless increase in density is largely due to the development of the MOS transistor in 1960, which became the cornerstone of modern microchips. This consistent growth in complexity has followed a trend described by Moore's Law, which predicted a doubling of transistor count roughly every two years. While it's been a powerful driver of innovation for over 50 years, the physical limits of miniaturization are increasingly evident. It remains to be seen if this rate of growth can be maintained given the challenges of quantum tunneling and nanoscale fabrication.
Integrated circuits are the brains behind many of our modern technologies. They powered the expansion of computing, enabling personal computers and cloud-based infrastructure. Their influence is also crucial in the world of telecommunications. The ability to squeeze a vast amount of processing power into smaller, more affordable devices has led to the rise of smartphones and pervasive mobile connectivity that connects billions. The economics of the semiconductor industry have grown to a massive scale, reaching into the trillions of dollars. The innovation and investments needed to push the boundaries of integrated circuits have huge ripple effects on global economies and geopolitical issues, particularly around supply chains.
The relentless drive for increased performance and miniaturization has sparked considerable research into new materials and fabrication techniques. EUV lithography and atomic layer deposition, along with other emerging nanostructuring methods, are crucial for creating transistors and other components smaller than the wavelength of visible light. This ability to manipulate matter at an incredibly small scale has had profound implications for device performance and energy consumption. This pursuit has also driven research into alternatives to traditional silicon-based technology, such as graphene and other 2D materials. Exploring new architectures like 3D integration and neuromorphic computing are other avenues being actively pursued.
The field is far from stagnant. Challenges in continued miniaturization and the potential consequences of quantum effects at the nanoscale necessitate a shift in design strategies. It remains to be seen if future innovation in integrated circuits will primarily rely on traditional transistor scaling or if a more radical departure towards new computing paradigms will be necessary. The relentless pace of integrated circuit development in the face of inherent physical constraints reflects the innovative spirit of this field and the profound impact these technologies will continue to have in shaping our future.
Revolutionize structural engineering with AI-powered analysis and design. Transform blueprints into intelligent solutions in minutes. (Get started for free)
More Posts from aistructuralreview.com: