Revolutionize structural engineering with AI-powered analysis and design. Transform blueprints into intelligent solutions in minutes. (Get started for free)
The Impact of Proper Foundation Grading on Structural Integrity A 2024 Analysis
The Impact of Proper Foundation Grading on Structural Integrity A 2024 Analysis - Geophysical Assessments Gain Prominence in Foundation Design Process
The role of geophysical assessments in foundation design is evolving, moving beyond a supplementary step towards a cornerstone of robust structural engineering. Methods like electrical resistivity and seismic surveys offer a powerful means of probing subsurface conditions, providing crucial information about the underlying geology that impacts a building's foundation. Understanding the nature of these subsurface conditions, especially in sites with diverse geological makeups, is critical. Integrating geophysical insights early in the design process helps engineers to anticipate and mitigate potential risks associated with unstable ground, ultimately contributing to longer-lasting, more resilient structures. This trend is further amplified by continuous advancements in foundation engineering techniques which demand ever more refined knowledge about the ground. These assessments not only guide the initial design phase but also support long-term monitoring and understanding of building performance and any subsequent degradation or changes. It's becoming clear that comprehensive geophysical evaluations are vital for building design, particularly for intricate projects that demand exceptional stability and safety.
In the evolving landscape of foundation design, geophysical assessments are assuming a more prominent role in ensuring structural integrity. These assessments, employing methods like electrical resistivity and seismic refraction, are crucial for understanding the subsurface conditions that underpin a structure's stability. Researchers have emphasized that overlooking the insights provided by geophysical data, especially in areas with heterogeneous soil conditions, can lead to inadequate foundation designs.
The integration of these techniques enables a more thorough understanding of geological features that can significantly influence foundation performance, such as the presence of fault lines or variations in rock layering. This detailed understanding can guide the selection of the most suitable foundation type, whether shallow or deep, thus preventing issues like excessive settlement. Moreover, these assessments can illuminate the presence and behavior of groundwater, which can have profound impacts on foundation performance and long-term stability.
Traditionally, foundation design has followed a three-phase approach, starting with preliminary concepts and estimations, progressing to detailed analysis, and culminating in implementation. However, with advancements in geophysical techniques, engineers can now incorporate real-time monitoring during construction. This dynamic approach facilitates immediate adjustments when unforeseen subsurface anomalies are detected, ultimately refining the construction process and enhancing the final structural integrity.
One could argue that the incorporation of geophysical assessments into foundation design is becoming increasingly necessary for several reasons, one being their ability to reduce unforeseen costs due to accurate subsurface predictions. In fact, the use of geophysical data can provide a more accurate view of the subsurface than traditional methods, reducing the chances of encountering unexpected challenges. Another benefit is the potential for increasing the service life of the structures built upon such foundations. Designs that are rooted in a complete understanding of subsurface variability are better equipped to withstand environmental forces. Finally, while the bearing capacity equation from Terzaghi (1943) continues to be foundational in evaluating shallow foundations, the advancement and integration of modern geophysical methods allow for much more detailed and comprehensive designs for modern infrastructure projects including bridges and tunnels. This trend, in turn, necessitates adjustments in engineering education, equipping future practitioners with the necessary skills to integrate geophysical data into their designs.
The Impact of Proper Foundation Grading on Structural Integrity A 2024 Analysis - Urban Bridge Substructure Reuse Challenges Traditional Grading Approaches
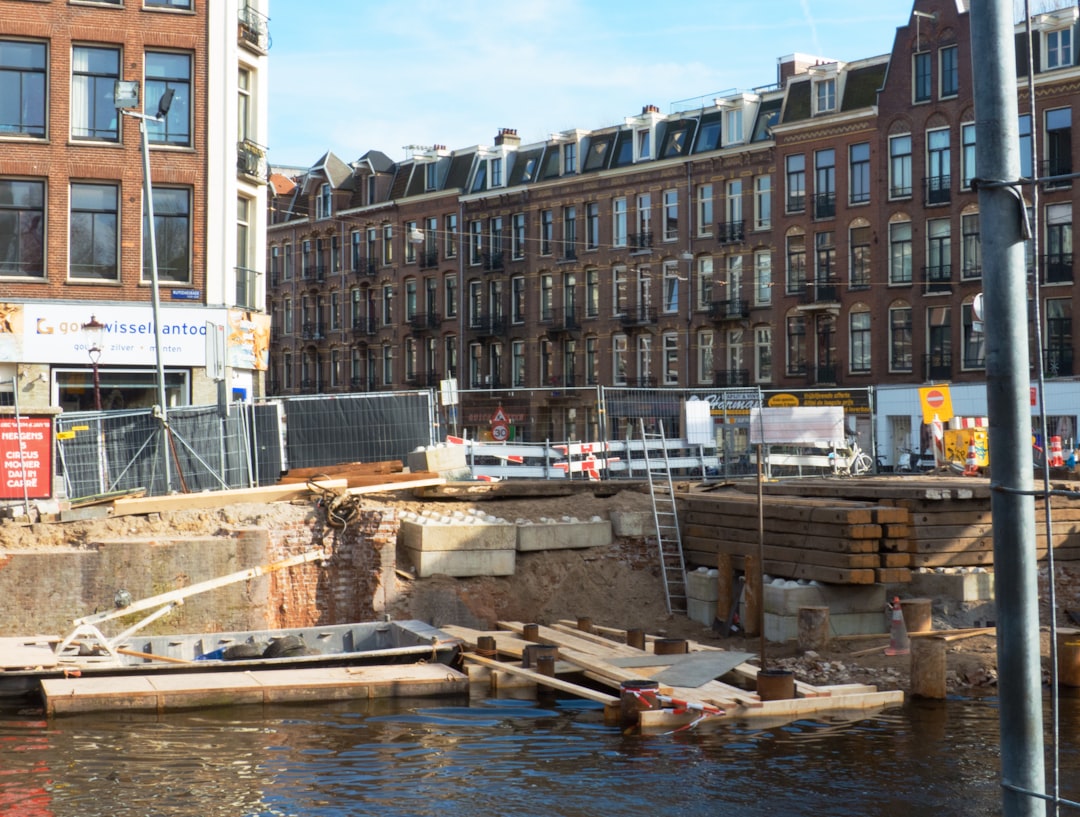
Reusing existing bridge substructures, especially in urban environments, presents challenges for traditional grading practices. While the reuse of bridge foundations offers economic and environmental benefits, particularly in areas with high infrastructure demands, it also introduces complexities. The variability and potential deterioration of older substructures require a more nuanced approach to grading compared to traditional methods. Traditional grading practices might not fully account for the unique characteristics and potential weaknesses of reused elements. Therefore, it's becoming crucial to incorporate advanced techniques, like utilizing seismic velocity readings, to thoroughly assess the structural integrity and load-carrying capacity of these reused components. This ensures that any necessary remediation work, such as grouting, is accurately targeted. As urban areas continue to evolve and bridge infrastructure needs change, adopting more comprehensive assessment methods for reused substructures is key to achieving the desired balance between cost-efficiency and long-term structural reliability.
The reuse of bridge foundations and substructures, particularly in urban areas undergoing bridge widening or replacement projects, presents a unique set of challenges for traditional grading approaches. This trend, while encouraged by organizations like the Federal Highway Administration (FHWA) for its economic, environmental, and traffic flow benefits, necessitates a rethinking of standard engineering practices. Many older bridge substructures were designed in an era before sophisticated geophysical techniques were widely employed, leading to potential discrepancies between the original design assumptions and the complexities of modern urban environments.
One key challenge stems from the significant variability of urban soil conditions. The lateral changes in soil density and stiffness are often not fully captured by traditional grading methods, potentially leading to underestimation of risks when reusing an existing substructure. Moreover, assessing the load-bearing capacity of reused components, especially when compared to current design standards and increased traffic loads, often reveals limitations in older designs. These discrepancies highlight the need for detailed assessments that go beyond standard grading practices.
The long-term stability of reused substructures can also be challenging to predict. Issues such as settlement, influenced by changing loads and aging materials, are often not easily addressed by conventional grading approaches. The rate of material degradation in bridge components can sometimes exceed expectations, raising serious concerns about the long-term structural integrity that traditional methods may not fully capture.
Furthermore, understanding how reused substructures respond dynamically to modern traffic and environmental conditions demands more advanced analytical tools than those typically employed in traditional grading. This need for dynamic response analysis reveals a gap in the traditional approach, especially for bridges that face a variety of modern environmental stressors.
The inherent complexities of urban settings also complicate the reuse process. Excavation work, a necessary part of bridge rehabilitation, can impose unexpected stresses on adjacent structures, a factor often overlooked in standard grading approaches. The lack of universally standardized practices and regulations related to bridge substructure reuse can also lead to inconsistencies in grading procedures and potential safety concerns, given the variation in interpretations across engineering disciplines.
Moving forward, incorporating modern sensor technologies that provide real-time data about stress and strain on reused components is crucial. These advanced sensors can highlight potential vulnerabilities that traditional grading methods might miss, emphasizing the need for a more adaptive and responsive approach to bridge engineering. This evolving field clearly necessitates a shift towards a more multidisciplinary perspective, bringing together geotechnical engineers, structural engineers, and urban planners to ensure that grading methods are well-suited for the challenges of reusing bridge substructures in modern cities. The average age of highway bridges in the US, with a significant percentage exceeding 50 years and a concerning portion in poor condition, reinforces the urgency and importance of addressing these challenges. Integrated Bridge Load Rating methods can help bridge the gap, but require a continued research and understanding of the complexities of reuse. It is apparent that moving forward, the industry needs to refine its understanding of the reuse of these structures, leveraging insights from workshops, research sessions, and improved guidelines.
The Impact of Proper Foundation Grading on Structural Integrity A 2024 Analysis - Seismic Considerations Reshape Foundation Grading Practices in Earthquake Zones
Seismic activity is forcing a reevaluation of how we approach foundation grading in earthquake zones. This shift emphasizes the crucial role of foundation design in ensuring structural integrity during seismic events. Understanding the complexities of soil behavior under seismic forces, combined with the variety of building types and foundation systems, necessitates a more nuanced approach to grading.
Proper grading practices are now seen as more than just a preliminary step; they are essential for distributing seismic loads effectively and maintaining structural stability. It's no longer sufficient to rely on traditional grading techniques. The industry is exploring advanced assessment methods, including those that utilize seismic data, to better understand how a foundation will behave during an earthquake. The goal is to design foundations that can not only withstand the anticipated ground movements but also minimize potential damage to the structure, enhancing safety within these vulnerable areas.
This evolving understanding is driving ongoing discussions amongst engineers and researchers about optimal solutions to design foundations in earthquake zones. The focus is on developing and implementing more innovative and effective strategies to meet these growing challenges.
Foundation grading in areas prone to earthquakes must acknowledge that ground shaking can modify soil behavior and cause sudden changes in lateral support. This makes relying solely on static analysis less reliable for ensuring a structure's integrity. Retrofitting existing structures for seismic resistance often requires reevaluating conventional grading practices, as they may not consider the dynamic loads induced by earthquakes, potentially leading to designs that are insufficient in areas with moderate to high seismic activity. Soil liquefaction, a phenomenon where waterlogged sand loses strength during earthquakes, can lead to severe failures in foundations with inadequate grading, further reinforcing the need for thorough assessments specifically in earthquake zones.
Employing advanced seismic grading methods can uncover unexpected shallow bedrock or hidden fault lines, significantly influencing foundation design choices and necessitating custom grading approaches. Research indicates that incorporating real-time seismic data into grading practices could significantly improve the precision of predictions for settlement and failure rates in areas prone to earthquakes, a departure from the usual methods found in conventional grading frameworks. It's noteworthy that even subtle alterations to foundation grading can substantially reduce seismic risks. For instance, slight adjustments to slope angles can enhance drainage and decrease soil pressure during an earthquake.
Modern grading practices are increasingly blending traditional soil mechanics with innovative geophysical technologies, establishing a more comprehensive approach to assessing seismic risks. However, a failure to adapt grading to seismic conditions can result in severe building performance issues, with research showing that poorly graded foundations can exacerbate ground motion effects, leading to increased potential damage. Many areas still utilize outdated grading codes that don't fully reflect the current scientific understanding of earthquake risks, highlighting a continuing need for updates in engineering standards. The interplay between grading practices and the local geology is so strong that, in certain regions, studies suggest that poor grading has contributed to a significant portion of structural failures during past earthquakes, emphasizing the need for incorporating seismic considerations into foundational design from the start.
The Impact of Proper Foundation Grading on Structural Integrity A 2024 Analysis - Microscopic Analysis Informs Macroscopic Structural Performance Predictions
Understanding how materials behave at the microscopic level is crucial for predicting how they'll perform on a larger, structural scale. This is especially relevant when considering the impact of foundation grading on overall structural integrity. Examining the makeup of materials at the microscopic level, particularly for composites, helps engineers understand how these tiny features affect the material's ability to withstand various stresses and loads.
Tools like multiscale modeling and Monte Carlo simulations allow engineers to bridge the gap between the extremely small and the large scale. These methods enable a more comprehensive understanding of how a material's behavior at the microscopic level leads to its macroscopic performance. This approach becomes particularly important when dealing with the challenges of reusing bridge substructures or designing foundations in areas susceptible to earthquakes. The ability to better predict material behavior using this microscopic-to-macroscopic approach enhances our capacity to understand potential weaknesses within these complex structures.
Furthermore, the integration of digital twins and real-time monitoring techniques is rapidly enhancing the accuracy of these predictions, leading to a more comprehensive understanding of the behavior of structures over time in varied and challenging environments. As our ability to analyze materials at smaller and smaller scales continues to improve, we can expect more accurate and robust designs that will ultimately improve the safety and lifespan of our infrastructure.
Understanding the microscopic world of soil and foundation materials can offer invaluable insights into how structures behave on a larger scale. The arrangement and characteristics of individual soil particles, like their size, shape, and the forces between them, significantly impact how the material responds to loads and stresses. For example, subtle variations in the type and composition of clay minerals at the microscopic level can dramatically influence the overall stiffness, plasticity, and compressibility of the foundation. This understanding is crucial for predicting how the foundation will behave under different loading scenarios.
The interactions between individual soil particles, whether through forces of cohesion, friction, or the development of slip planes, can predict larger-scale phenomena like settlement and sliding. This emphasizes the importance of understanding granular mechanics in foundation design, especially in areas prone to seismic activity. In a similar vein, new developments in concrete-like materials, such as geopolymers, are better understood through observation of their microstructure. This microscopic analysis allows engineers to tailor their design, aiming for enhanced durability and load-bearing capacity within the foundation.
Microscopic examination can also serve as an early warning system for potential failure. By detecting microcracks within concrete or soil, predictive modeling of structural failures becomes possible. Such insights can lead to preventive measures, such as strategically placed reinforcement, thereby enhancing the overall structural integrity of the built environment. Furthermore, the intricate pore spaces within soil, visible at a microscopic level, heavily influence the flow of water. Understanding the hydraulic conductivity within these pore spaces is vital, particularly in foundational contexts susceptible to fluctuating water levels. This understanding is critical to managing risks associated with erosion, liquefaction, or altered drainage patterns.
Even temperature variations can alter the behavior of foundational materials at the microscopic level. This can result in observable expansion or contraction at a larger scale, influencing the stability of the structure, particularly in regions with extreme temperature shifts. Improving the soil itself, through techniques such as densification or grouting, can also be optimized with the help of microscopic analysis. Examining how these treatments change the structure of the soil allows engineers to design foundations with improved load-bearing capabilities and reduced settlement risk. The advancement of non-destructive testing technologies, employing microscopic techniques, provides engineers with opportunities for real-time monitoring of internal structural conditions. This may allow for the early detection of weaknesses before they result in observable failures, improving both safety and efficiency.
Finally, microscopic analysis allows researchers to track the degradation of materials over time. By understanding how materials change at a microscopic level when exposed to environmental factors, we can better estimate the rate of deterioration in foundational elements. This information helps in long-term maintenance planning and the development of more durable materials for future projects. The application of microscopic analysis to foundation materials and structures has the potential to refine our understanding of how seemingly small changes can impact the overall structural performance, and this understanding will be crucial for ensuring the long-term safety and resilience of future construction projects.
The Impact of Proper Foundation Grading on Structural Integrity A 2024 Analysis - Long-Term Lifecycle Impacts of Foundation Grading on Structural Integrity
The long-term performance and safety of a building are intrinsically tied to the quality of its foundation grading. While initial grading aims to prevent immediate issues like water damage and initial settling, its impact extends far beyond the construction phase. Proper grading practices establish a strong foundation that can withstand the cumulative effects of environmental factors, usage patterns, and the natural degradation of materials over time. Neglecting appropriate grading can lead to a cascade of problems, including the appearance of cracks, which, if not addressed, can escalate into significant structural failures and safety concerns, demanding costly repairs and potentially impacting the lifespan of the structure.
Beyond the macroscopic impact, a deeper understanding of the materials used in the foundation, especially at the microscopic level, allows for more precise predictions of how those materials will react over time. This is particularly crucial when facing challenging environmental conditions, like temperature shifts or seismic activity, that can induce stresses on the foundation. Incorporating modern technologies, such as digital twin models and real-time monitoring systems, into the design process enhances our ability to anticipate and manage these changes. This integration of advanced technology, coupled with a thorough understanding of material behavior at all scales, creates a framework for optimizing the long-term structural integrity of buildings. By focusing on the long-term impacts of initial grading decisions, we can significantly increase the chances of a building remaining safe and structurally sound for decades to come.
1. **Foundation Grading's Influence on Long-Term Deformation:** How a foundation is graded significantly influences how a structure deforms over time. If the grading isn't done well, it can lead to uneven settling and persistent warping, which impacts a building's structural integrity and how loads are distributed.
2. **Soil Liquefaction and Grading:** Traditional grading methods often don't sufficiently consider the potential for soil liquefaction in areas with water-saturated, loose soil during earthquakes. Inadequate grading in earthquake-prone zones can make foundations very unstable and cause catastrophic failures.
3. **Historical Evidence for Effective Grading:** Looking at how buildings have performed over time reveals that those with properly graded foundations usually need less maintenance and repair in the long run. This highlights the link between good grading and preventing structural problems over a building's lifespan.
4. **Microscopic Changes in Soil Under Load:** Over time, soil under consistent pressure experiences changes at the microscopic level, which can affect its strength. Using microscopes to study these changes can help predict how a foundation will perform in the future.
5. **Sensitivity of Structures to Deflection:** A building's structural integrity can be very sensitive to any deflection or movement caused by poor grading. Even small amounts of deflection can accumulate, leading to substantial weaknesses and failures that can occur far later than initially expected.
6. **Long-Term Drainage Problems Related to Grading:** The design of how a foundation is graded often doesn't consider how drainage will work long-term. If grading is poor, water can build up, potentially causing soil erosion, instability, and impacting the overall structural integrity.
7. **Impact of Geological Variations on Grading:** The characteristics of the soil and ground water in a particular location can significantly influence how well a graded foundation performs over time. In places where the soil properties change a lot, using a one-size-fits-all grading approach often results in structural problems.
8. **Thermal Expansion and Foundation Grading:** The way a foundation is graded can affect how the foundation materials expand and contract due to temperature changes. Fluctuations in temperature can cause expansion and contraction, and poor grading can exacerbate these effects, leading to cracking and displacement in joints.
9. **Foundation Grading and Buried Infrastructure:** The long-term stability of underground utilities and infrastructure is directly tied to how the foundation is graded. Poor grading can cause shifting or buckling of these systems, leading to failures and expensive repairs.
10. **Ongoing Monitoring and Adaptive Strategies for Foundations:** Because foundation issues can take decades to develop, it's essential to keep an eye on how graded foundations perform. Advanced sensors can provide real-time information about changes, allowing engineers to adjust maintenance and repair plans proactively to keep the structural integrity sound over time.
Revolutionize structural engineering with AI-powered analysis and design. Transform blueprints into intelligent solutions in minutes. (Get started for free)
More Posts from aistructuralreview.com: