Revolutionize structural engineering with AI-powered analysis and design. Transform blueprints into intelligent solutions in minutes. (Get started for free)
Unveiling the Distinct Mechanisms Conduction vs
Convection in Heat Transfer
Unveiling the Distinct Mechanisms Conduction vs
Convection in Heat Transfer - Defining Conduction and Convection in Heat Transfer
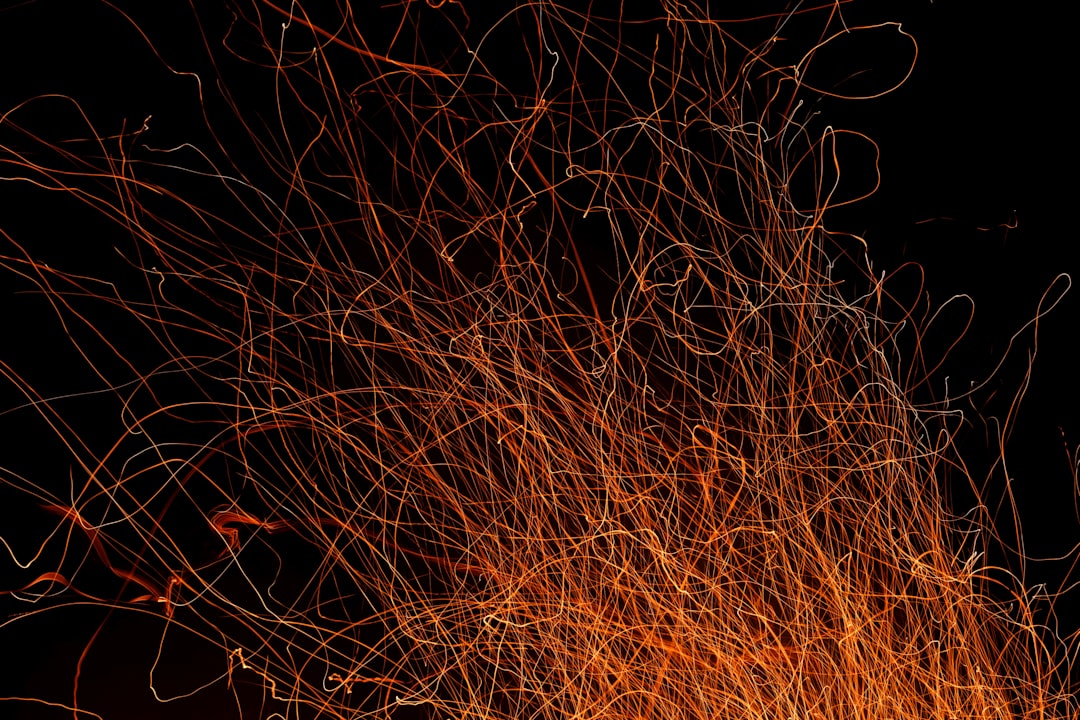
Heat transfer, the movement of thermal energy due to temperature disparities, relies on various mechanisms. Conduction and convection represent two primary methods through which this transfer occurs. In conductive heat transfer, energy moves through a material by direct contact between its particles. This is most prevalent in solids and heavily depends on a material's intrinsic ability to conduct heat, often referred to as its thermal conductivity. In essence, heat flows from hotter regions to colder regions within the material itself.
Convection, on the other hand, involves the movement of fluids—liquids or gases—as the primary means of heat transfer. This motion can be driven by natural forces, like buoyancy caused by temperature differences within the fluid, or by external forces like fans or pumps, termed forced convection. Essentially, the fluid acts as a carrier, transporting heat energy from one location to another. The behavior of both natural and forced convection hinges on the fluid's properties and the specific conditions within the system.
The distinct characteristics of conduction and convection highlight the intricate nature of heat transfer. Comprehending these differences is paramount when analyzing and designing thermal systems across numerous applications. Understanding how these distinct mechanisms operate within various contexts is fundamental to effectively managing and optimizing heat transfer in a wide range of fields.
1. We can differentiate conduction and convection by considering the medium through which heat travels. Conduction primarily occurs within solids, relying on direct molecular interactions. In contrast, convection involves the movement of fluids—liquids or gases—carrying heat energy along with them.
2. The efficiency of conductive heat transfer is intrinsically linked to a material's thermal conductivity. This property varies greatly between different substances. Highly conductive materials, like copper, readily facilitate heat flow, while substances like wood are considerably less efficient at conduction.
3. Convection, in its diverse forms, includes natural convection, driven by density differences arising from temperature variations within the fluid. Then there's forced convection, where external influences like pumps or fans induce the fluid movement.
4. The mathematical description of conduction is based on Fourier's Law, which highlights the relationship between heat flux and the temperature gradient. Convection, on the other hand, is often described using Newton's Law of Cooling, reflecting a different underlying mechanism.
5. When it comes to effectiveness, convection surpasses conduction in heat transfer. Consider heating water: boiling through convective currents is much faster than the conduction that would occur solely through the pot's base.
6. Factors such as the fluid's speed, the surface area exposed, and the temperature difference play a significant role in the efficiency of convection. These interactions make analyzing convective systems, such as HVAC, quite complex, but crucially important for energy-efficient designs.
7. Conduction can still occur in a vacuum—albeit through radiation or virtual particle interactions. Conversely, convection demands a fluid medium and cannot happen in a vacuum's void.
8. The flow regime, either turbulent or laminar, significantly alters convective heat transfer. Turbulent flow enhances heat transfer due to its increased mixing, contrasting with laminar flow, where layers can separate and obstruct efficient heat movement.
9. In engineering, understanding both conduction and convection is fundamental for managing thermal systems effectively. Errors in analysis can lead to problematic overheating and potential failure in crucial components like engines or electronics.
10. The interplay between conduction and convection is central to the operation of heat exchangers. Conduction facilitates heat transfer through solid materials, while convection manages the transfer between the fluids in contact. This combined process allows for optimization in various industrial thermal operations.
Unveiling the Distinct Mechanisms Conduction vs
Convection in Heat Transfer - Molecular Mechanisms Behind Conduction
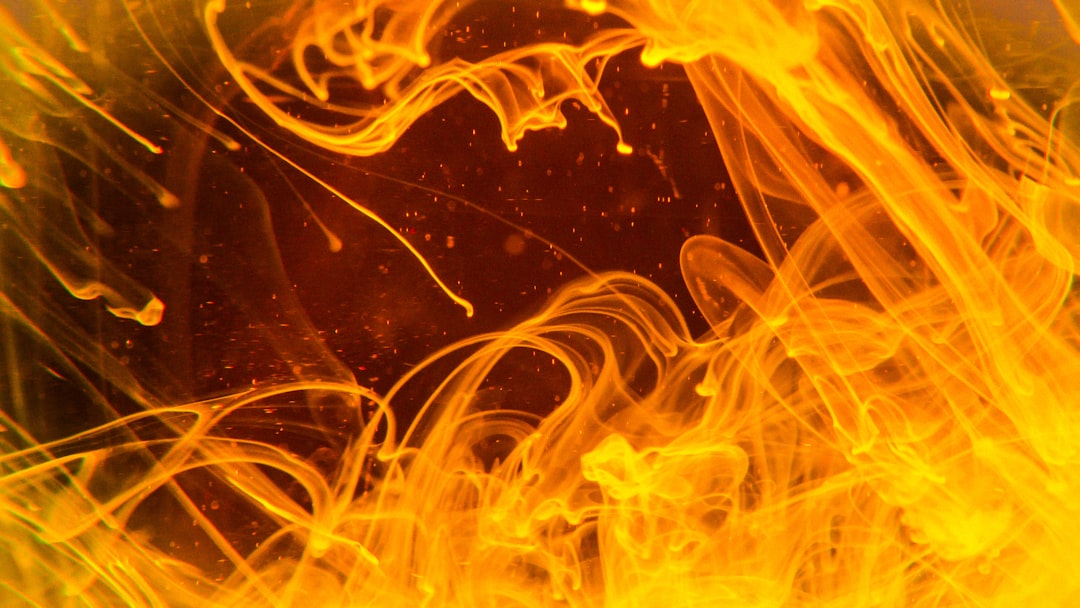
At the heart of conduction lies a fascinating interplay of molecular interactions that govern the flow of thermal energy through solids. This process is driven by the kinetic energy of molecules, where regions of higher temperature, possessing molecules with greater kinetic energy, transfer energy to cooler regions with less molecular motion. This transfer, unlike convection's reliance on fluid movement, relies solely on direct contact between molecules. The efficiency of this transfer is intimately tied to the material's inherent ability to conduct heat, known as thermal conductivity. This property varies significantly between materials, affecting how readily they transfer thermal energy. Consequently, understanding these molecular interactions is crucial in applications where precise thermal control is paramount, like in engine design or electronic components. The understanding of how these molecular mechanisms influence the flow of heat is fundamental to optimizing heat transfer across many engineering disciplines.
1. The effectiveness of conduction hinges on factors like the material's microstructure, specifically its grain structure and the presence of impurities. For instance, metals with a more ordered, crystalline structure tend to be better heat conductors compared to those with more defects, which disrupt the flow of heat.
2. The speed at which heat travels through a conductive material can be tied to the average distance particles travel before colliding—the mean free path. This distance isn't fixed, it can vary depending on both temperature and the material's composition, ultimately impacting the overall conductivity.
3. Phonons, quantized vibrational waves within a crystal lattice, play a significant role in heat conduction in solids. Their behavior and ability to facilitate energy transfer under specific conditions are crucial for understanding the nature of thermal conductivity.
4. Although we often think of conduction as primarily a solid-state phenomenon, it can also occur in liquids and even gases, but to a much lesser degree. In these cases, the heat transfer relies on molecular collisions, but the efficiency is considerably lower compared to solids.
5. It's important to acknowledge that the thermal conductivity of a material isn't constant—it changes with temperature. This is a key aspect in engineering. For example, many metals show improved conductivity when heated, while certain insulating materials might exhibit better properties at lower temperatures.
6. The concept of thermal resistivity provides a useful counterpoint to conductivity. Materials with low thermal conductivity inherently have high thermal resistivity. This characteristic is particularly relevant in managing heat in engineering applications like electronic device cooling.
7. Investigating the roles of the mean free path and phonon scattering in different materials could lead to advances in fields like nanotechnology. Researchers can potentially engineer materials at the molecular level to enhance specific conductive properties for targeted applications.
8. Conduction is inherently a local process: heat transfer only occurs between particles that are directly adjacent to one another. As a result, even a slight temperature difference can lead to substantial heat transfer rates in materials that are highly conductive.
9. Some nanostructured materials exhibit rather unusual heat conduction behavior, such as ballistic conduction. In this phenomenon, phonons can travel further distances without scattering, deviating from the typical predictions of classical conduction theory.
10. Exploring the mechanisms behind heat conduction has spurred important advancements in materials science. Researchers are developing metamaterials designed to manipulate thermal conductivity. This work has the potential to generate innovative heat management solutions across a wide array of fields.
Unveiling the Distinct Mechanisms Conduction vs
Convection in Heat Transfer - Fluid Dynamics in Convective Heat Transfer
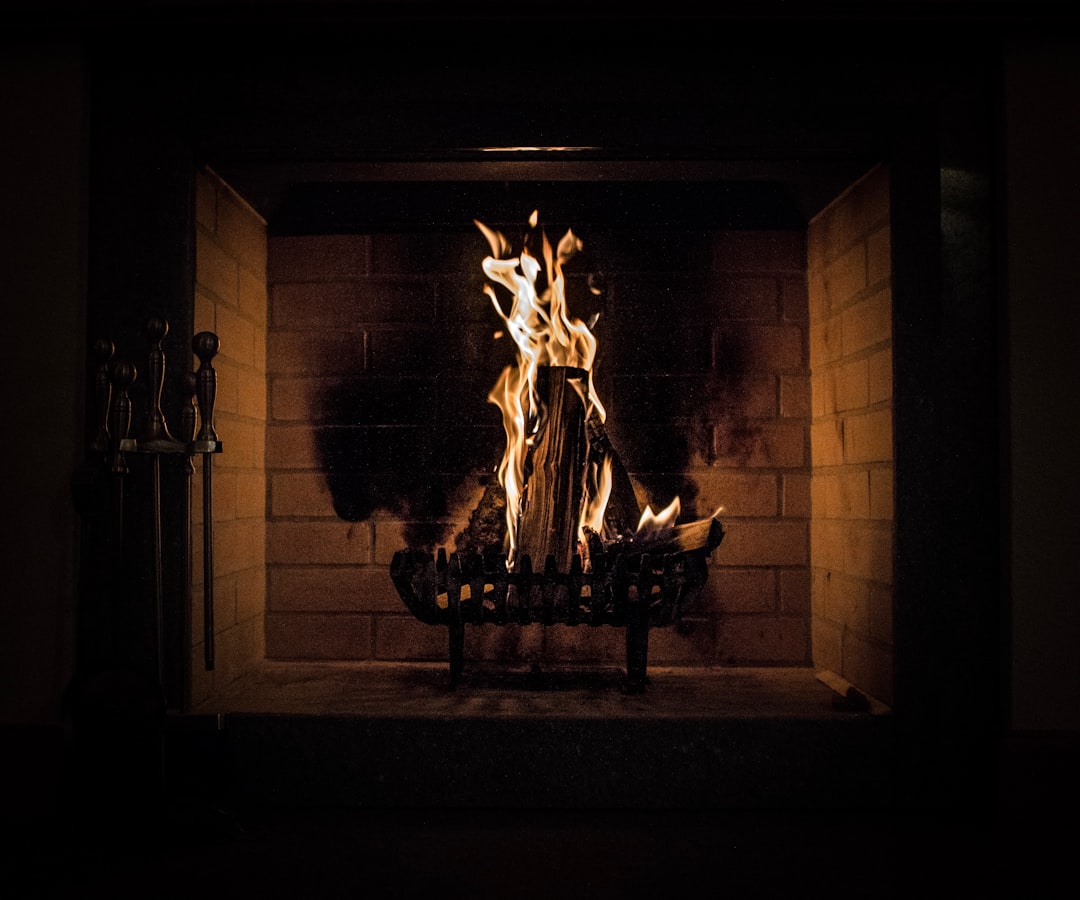
Fluid dynamics is essential in understanding how convection transfers heat. Convection relies on the motion of fluids, whether liquids or gases, to carry thermal energy. This movement can be natural, driven by density differences caused by temperature variations (natural convection), or forced through external mechanisms like fans or pumps (forced convection). The rate at which heat is transferred through convection is heavily influenced by aspects like the speed of the fluid, the surface area involved, and the temperature differences within the system. This interconnectedness makes understanding fluid dynamics critical for optimizing any system that relies on convective heat transfer.
It's particularly important to consider the nature of fluid flow itself – whether it's smooth and layered (laminar) or chaotic and mixed (turbulent). Turbulent flow tends to be more effective at heat transfer due to its increased mixing of the fluid, whereas laminar flow can sometimes impede the process. This understanding is crucial in the design of a variety of applications, from the everyday, like heating and cooling systems (HVAC), to more specialized areas like managing the heat generated in electronic devices. The principles of fluid dynamics form the basis for efficiently managing and controlling thermal energy within these systems.
1. **Flow's Impact on Heat Transfer**: The rate at which heat is transferred through convection isn't solely dependent on the fluid's temperature. It's also heavily influenced by how the fluid moves, particularly the flow pattern itself. The Reynolds number, for example, is a vital parameter that can help us predict if the flow will be smooth (laminar) or chaotic (turbulent), and this directly affects heat transfer.
2. **Nusselt Number's Role**: The Nusselt number (Nu) is a critical dimensionless quantity in convection. It essentially tells us how much better heat is transferred through convection compared to conduction alone. A higher Nusselt number indicates that the fluid's movement is enhancing heat transfer, which can be useful information for design.
3. **Gravity's Influence**: In natural convection, gravity plays a crucial role in setting up the flow. Because of density differences caused by temperature variations, heated fluid tends to rise and cooler fluid falls. This means that even a slight change in the orientation of a fluid system can significantly affect how heat is transferred through it.
4. **Heat Transfer Coefficient's Variability**: The heat transfer coefficient, a key parameter representing the efficiency of convective heat transfer, is far from constant. It's influenced by the flow conditions. For instance, if we increase the flow velocity in a forced convection situation, the heat transfer coefficient generally increases in a non-linear fashion, leading to a higher overall heat transfer rate.
5. **Phase Changes**: When a fluid changes phase, like boiling or condensation, convective heat transfer can become significantly more efficient. The heat involved in these phase transitions, called latent heat, significantly boosts the heat transfer rate, often outpacing standard convective contributions.
6. **Surface Roughness Matters**: The texture of a surface can influence convection. Rough surfaces can promote turbulence near the surface, resulting in better heat transfer compared to smooth surfaces where a more stable, laminar boundary layer might form.
7. **Critical Heat Flux**: In the context of boiling, there's a crucial point called the critical heat flux. If we exceed this threshold, the heat transfer mechanism shifts drastically from efficient boiling to a very inefficient type of boiling called film boiling, potentially causing issues with overheating.
8. **Importance of Fluid Properties**: Different fluids have different properties—viscosity, density, specific heat, and so on—that interact in complex ways to affect heat transfer. These factors play a vital role in the performance of thermal systems, making the choice of working fluid a key decision.
9. **Dealing with Two-Phase Flow**: When both gas and liquid phases are present (two-phase flow), analyzing convective heat transfer becomes much more complicated. The intricate interplay between the phases has a major impact on the overall heat transfer process and needs to be taken into account in designs like heat exchangers and cooling systems.
10. **Computational Tools**: Today's engineers increasingly use computational fluid dynamics (CFD) to model and predict complex convective heat transfer situations. CFD provides a way to simulate and visualize complex flow patterns and temperatures, helping designers make informed choices for thermal management systems.
Unveiling the Distinct Mechanisms Conduction vs
Convection in Heat Transfer - Natural vs Forced Convection Processes
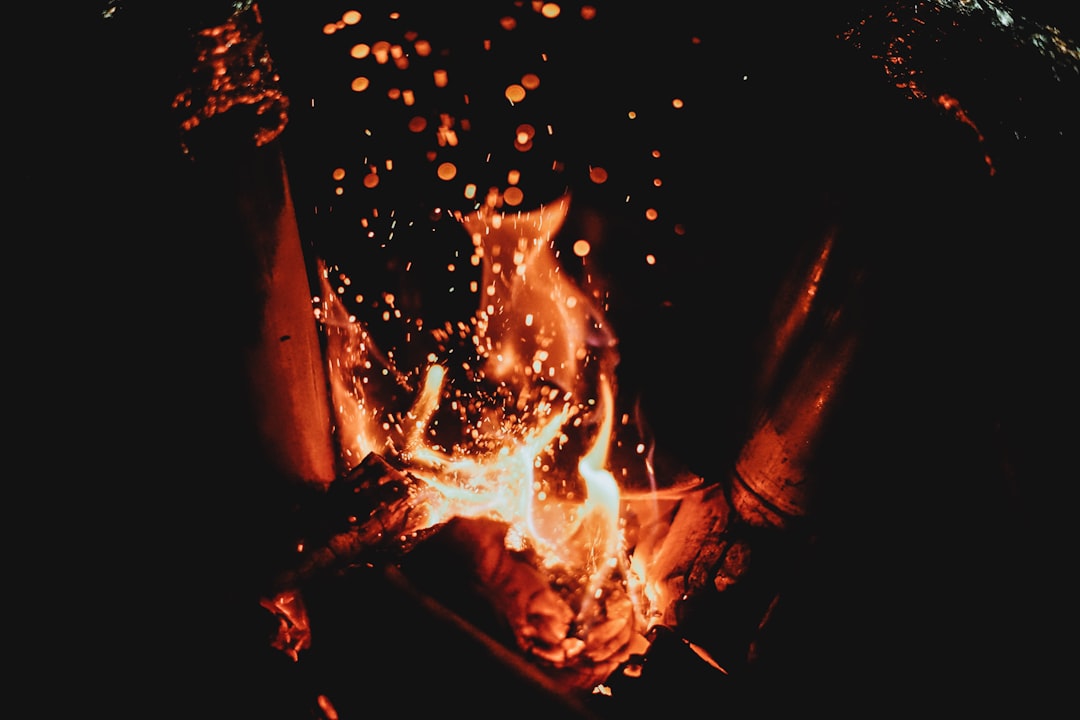
Natural and forced convection represent two fundamental ways heat transfers through fluids. In natural convection, fluid movement is driven by density differences that result from temperature variations. Warmer, less dense fluid rises, while cooler, denser fluid sinks, creating a natural circulation pattern. This process happens spontaneously, without any external force. On the other hand, forced convection employs external devices like fans or pumps to actively move the fluid. This enforced movement considerably accelerates heat transfer compared to natural convection.
The effectiveness of both processes hinges on various factors, including the fluid's inherent properties, the speed at which it moves, and the temperature difference across the system. For example, in a room heated by a radiator, the rising warm air represents natural convection, while the circulation facilitated by a fan would be forced convection. Understanding how these processes differ is vital for managing heat transfer efficiently in a wide array of applications, from designing HVAC systems to cooling electronic devices. Choosing between natural and forced convection depends on the specific situation, with factors like desired heat transfer rate and system constraints influencing the optimal choice.
1. **Natural convection's inherent variability** stems from its reliance on subtle temperature differences to generate buoyancy forces. This can lead to less predictable flow patterns, particularly in enclosed environments, making it harder to analyze heat transfer accurately.
2. **Forced convection's ability to control heat transfer** surpasses natural convection due to its use of external forces like fans or pumps. This control extends to influencing the flow regime, potentially shifting from laminar to turbulent flow, resulting in a significant increase in heat transfer efficiency.
3. **Buoyancy forces driving natural convection** are highly sensitive to fluid temperature changes. Even small shifts in temperature can cause significant alterations in flow patterns, leading to fluctuations in the overall heat transfer rate within the system.
4. **Surface orientation plays a crucial role** in natural convection processes. The flow behavior and, thus, heat transfer rates can differ greatly between horizontal and vertical surfaces due to how gravity influences the buoyant forces acting on the fluid.
5. **Phase change scenarios see enhanced heat transfer** under forced convection. Processes like boiling dramatically increase heat transfer rates due to the significant contribution of latent heat, often surpassing standard convective heat transfer by orders of magnitude.
6. **The Reynolds number provides valuable insights** not only into whether flow is laminar or turbulent, but also on how it affects heat transfer characteristics. In forced convection designs, engineers can use the Reynolds number to achieve a desired system performance by fine-tuning fluid velocity.
7. **Thermal boundary layers form near surfaces** in both natural and forced convection, restricting heat transfer due to the fluid's viscosity. The thickness of these layers is dynamic and depends on the fluid's velocity and the temperature difference, highlighting the complex and interconnected nature of heat transfer in these cases.
8. **Fluid properties like viscosity and density** are significant factors impacting both natural and forced convection. Even a slight temperature change can alter these properties, leading to subsequent changes in the overall heat transfer coefficient of the system.
9. **Design choices must consider both convection types** in systems like heat exchangers where the interplay between natural and forced convection significantly influences efficiency. Failing to accurately predict flow characteristics in these cases can lead to design inefficiencies and potentially overheating.
10. **Computational fluid dynamics (CFD) modeling** is helpful for predicting convective heat transfer, but accurately capturing the interplay of turbulence, fluid properties, and heat transfer remains a challenging endeavor. This necessitates careful validation of CFD results against experimental data for reliable and accurate predictions in real-world applications.
Unveiling the Distinct Mechanisms Conduction vs
Convection in Heat Transfer - Factors Affecting Conduction and Convection Rates
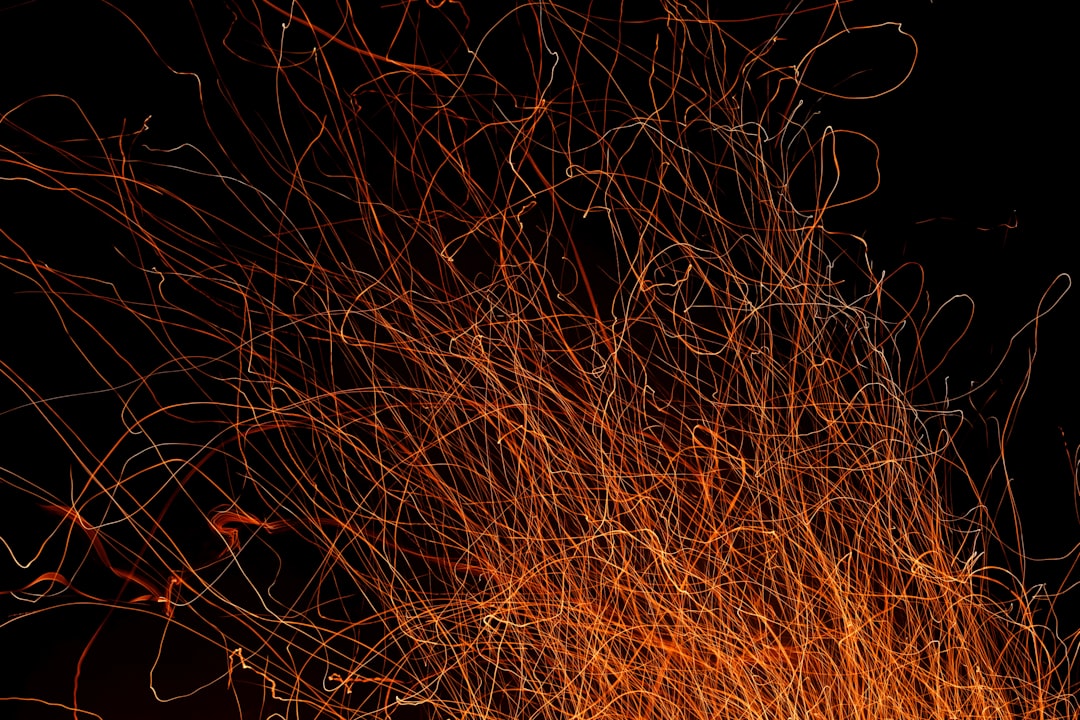
The speed at which heat is transferred through conduction and convection, two primary methods of heat transfer, is affected by a number of factors. For conduction, these include the material's inherent ability to conduct heat (thermal conductivity), the size of the area where heat is being transferred, and the difference in temperature between the areas. Convection's efficiency is similarly impacted, with factors such as the properties of the fluid (like its thickness and density), how the fluid is moving (its flow dynamics), and the temperature variation in the system being key considerations. When convection is natural, driven by differences in the fluid's density caused by temperature, predictions become more difficult as the flow patterns can be less predictable due to temperature-induced density shifts. However, when convection is forced, using mechanisms such as pumps to move the fluid, the heat transfer rates can increase dramatically as the flow conditions can be more precisely controlled, often leading to more turbulent flow. Recognizing how each of these variables influences heat transfer is vital for effectively designing thermal systems in a range of engineering disciplines.
1. **Temperature Differences and Convective Flow**: The rate of convective heat transfer is profoundly impacted by the temperature difference within the system. A larger temperature variation leads to stronger driving forces, resulting in faster fluid motion and ultimately a higher rate of heat transfer. This emphasizes the importance of carefully controlling temperature gradients for optimal convective heat transfer.
2. **Material Thickness and Conduction Resistance**: The thickness of a material significantly influences its effectiveness as a heat conductor. Thicker materials offer a greater distance for heat to traverse, increasing the chances of energy loss through mechanisms like radiation or heat leakage from the material's surfaces. This factor is particularly relevant when considering materials for thermal insulation or heat sinks in engineering applications.
3. **Density Fluctuations in Natural Convection**: Density differences play a crucial role in natural convection. Even small variations in fluid density due to temperature changes can generate significant shifts in the natural circulation patterns. Understanding the relationship between temperature and density is crucial for predicting and controlling heat transfer in situations where natural convection dominates.
4. **Material Purity and Conduction**: The presence of impurities or defects within a solid material can negatively impact its ability to conduct heat. These imperfections can disrupt the lattice structure and interfere with the efficient transfer of vibrational energy through the material. This highlights the importance of material purity and structural uniformity in applications where effective heat conduction is crucial.
5. **Reynolds Number and Flow Regime**: The Reynolds number is a critical dimensionless parameter in fluid dynamics that helps us understand the flow regime (laminar or turbulent). Turbulent flow, characterized by chaotic fluid mixing, leads to significantly enhanced heat transfer compared to laminar flow. Understanding this distinction is vital for maximizing heat transfer in systems where fluid motion is the dominant mechanism.
6. **Viscosity and Temperature**: The viscosity of a fluid, a measure of its resistance to flow, is often heavily influenced by temperature. As temperature increases, fluid viscosity typically decreases, which can facilitate easier fluid movement and enhance convective heat transfer. This relationship is important when selecting working fluids for specific thermal systems, especially when considering the impact of varying operating temperatures.
7. **Predictability Challenges in Natural Convection**: Natural convection, relying on density differences within a fluid, can lead to complex and unpredictable flow patterns, especially in enclosed spaces. This makes predicting heat transfer in such systems more challenging than in cases where forced convection is used. Accurately modeling and understanding these complex flow patterns is a persistent challenge in thermal engineering.
8. **Surface Area and Heat Exchange**: The surface area in contact with a fluid directly impacts the rate of heat transfer in both conduction and convection. Larger surface areas provide more opportunities for interaction between the solid surface and the fluid, increasing the potential for heat exchange. This understanding is fundamental in optimizing the design of heat exchangers and other systems where surface area plays a key role in thermal performance.
9. **Critical Heat Flux and System Safety**: In boiling situations, exceeding the critical heat flux can dramatically shift the heat transfer mechanism from an efficient, nucleate boiling state to a less effective film boiling state. This transition can lead to overheating and safety concerns in thermal systems. Hence, understanding and avoiding the critical heat flux is paramount in engineering design to maintain proper operating conditions and prevent failure.
10. **Nanofluid Technology**: Recent research explores the use of nanofluids—liquids containing nanoparticles—to enhance heat transfer. The addition of these particles can significantly increase the thermal conductivity of the base fluid, improving the effectiveness of both conduction and convection. This promising field has the potential to revolutionize thermal management in various applications where efficient heat removal or transfer is crucial.
Unveiling the Distinct Mechanisms Conduction vs
Convection in Heat Transfer - Practical Applications of Conduction and Convection
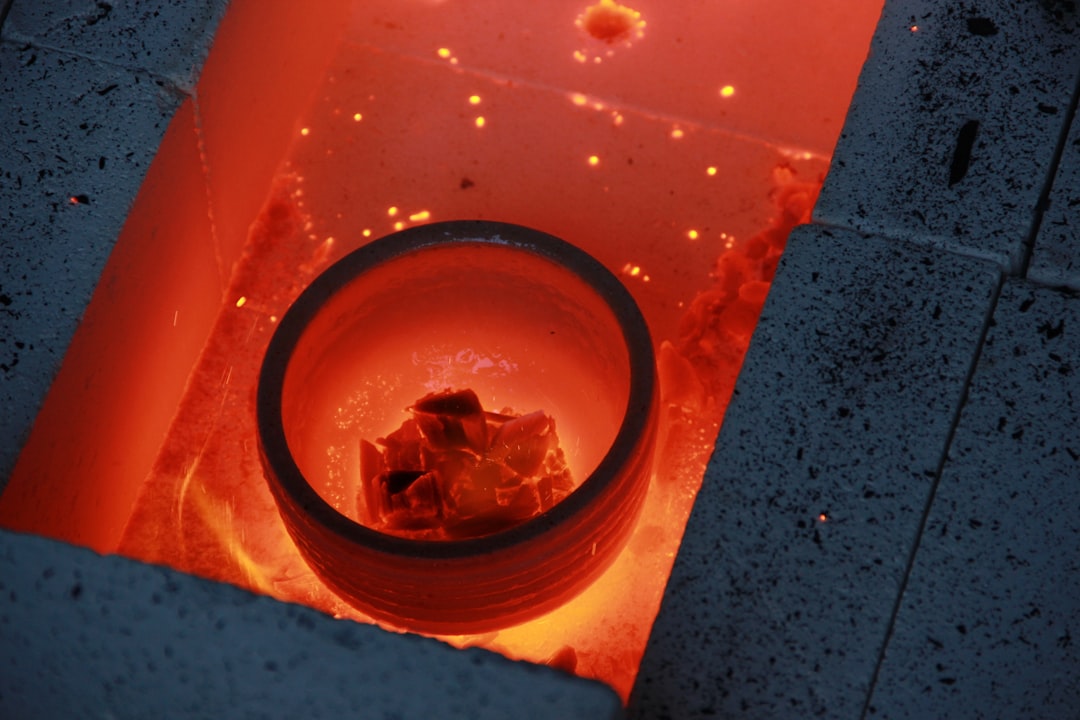
The practical applications of conduction and convection are widespread and essential across various fields, impacting everything from everyday experiences to sophisticated engineering systems. Conduction, the transfer of heat through direct contact within a material, finds vital applications in areas like thermal insulation, heat sinks designed to dissipate heat from electronic components, and the management of heat in various structures and devices. On the other hand, convection, relying on fluid movement to transfer heat, forms the foundation of systems like heating, ventilation, and air conditioning (HVAC), where efficient heat transfer relies on either natural fluid circulation or forced movement by external devices. The interplay between these two mechanisms presents both opportunities and challenges in design and engineering. Achieving optimal performance in systems that rely on both often requires a delicate balance of conduction and convection, which can lead to innovative, energy-efficient designs. A thorough understanding of these heat transfer processes provides a framework for improved design decisions and helps to optimize the safety and effectiveness of a diverse range of thermal systems. While beneficial in their own right, the individual and combined use of these mechanisms also reveal some shortcomings that can impede efficiency, and thus are worthy of deeper critical analysis. For example, systems relying on solely natural convection can exhibit unpredictability, while those focused on forced convection may require excessive energy. Addressing these constraints can improve system efficiency and reliability in applications across various industries and facets of life.
1. **Temperature Differences Impacting Conduction**: The rate at which heat conducts through a material can be dramatically influenced by the temperature gradient within that material. For instance, if one end of a metal rod is heated significantly more than the other, heat will flow more rapidly compared to a uniformly heated rod. This reveals the close relationship between temperature disparities and the speed of conductive heat transfer.
2. **Surface Geometry's Role in Convection**: The shape of surfaces interacting with a fluid significantly affects convective heat transfer rates. Irregular or rough surfaces are more likely to promote turbulent flow, which generally enhances heat exchange compared to smooth surfaces where a laminar flow layer often develops.
3. **Critical Heat Flux Variability Across Systems**: In situations involving boiling or phase changes, the critical heat flux (CHF), which represents the maximum heat transfer rate achievable before the transition to inefficient boiling, isn't solely dependent on the fluid itself. Surface texture and orientation can play a role in determining this critical value, leading to variability that's crucial to understand in systems like electronics cooling.
4. **Convective Boundary Layer Effects**: In both natural and forced convection, thermal boundary layers develop near surfaces, which hinder efficient heat transfer due to the fluid's viscosity. The characteristics of these layers, such as their thickness, depend strongly on fluid properties and flow regime, underscoring the need to understand and potentially manage them in engineering designs aiming for optimal heat transfer.
5. **The Prandtl Number's Relevance**: The Prandtl number, a dimensionless quantity that signifies the ratio between momentum and thermal diffusivity in a fluid, provides a vital understanding of the connection between the fluid's dynamic behavior and its ability to conduct heat. It's essential for accurately predicting heat transfer rates in both conduction and convection, especially when facing complex flow situations.
6. **Velocity, Turbulence and Temperature in Forced Convection**: In forced convection scenarios, increasing the velocity of a fluid increases turbulence and generally leads to enhanced heat transfer rates. But, it's important to recognize that a higher fluid velocity can also create steeper temperature gradients in the flow, leading to some non-intuitive relationships between flow rate and overall heat transfer efficiency.
7. **Graphene's Remarkable Thermal Conductivity**: Certain materials, like graphene, exhibit incredibly high thermal conductivity, facilitating efficient heat transfer through conduction. This unique characteristic, linked to graphene's unusual structure, offers a path towards tackling thermal challenges in systems like electronic devices at the nanoscale. This area of research holds potential for advancements in microelectronics cooling.
8. **Specific Heat Capacity and Heat Transfer**: The specific heat capacity of a fluid—how much heat energy it takes to raise its temperature—plays a key role in its heat transfer capacity. Fluids with high specific heat capacities can absorb and store more thermal energy, making them more efficient in cooling applications where the ability to soak up heat is crucial.
9. **Dielectric Fluids and Electronics Cooling**: In the realm of electronics cooling, dielectric fluids have become increasingly important because they can prevent short circuits and transfer heat via both conduction and convection. These materials are potentially well-suited for cooling electronic systems that require careful protection against short circuits or potential dielectric breakdown.
10. **Nanofluids and Enhanced Heat Transfer**: Nanofluids, composed of nanoparticles suspended in a liquid, can significantly impact thermal properties and heat transfer rates. Research indicates that these fluids can exhibit increased thermal conductivity compared to the base liquid, suggesting that they hold promise for cooling systems, heat exchangers, and other thermal management applications where advanced performance is necessary.
Revolutionize structural engineering with AI-powered analysis and design. Transform blueprints into intelligent solutions in minutes. (Get started for free)
More Posts from aistructuralreview.com: